by Dr. Alec MacAndrew (20 October 2014)
That Shepherd, who first taught the chosen Seed
In the Beginning how the Heav’ns and Earth
Rose out of Chaos – John Milton
If life is going to exist in a Universe of this size, then the one thing it cannot
afford to have is a sense of proportion… – Douglas Adams
1 Introduction
The New Geocentrists have been claiming for some time that recent measurements of certain cosmological features “point straight at the Earth”[1], and that therefore the Earth must be in the centre of the Universe. More recently, they have been promoting their movie, The Principle, which they hype as “one of the most controversial films of our time”[2]. The movie is ostensibly about a “fair, balanced and comprehensive treatment” of the Copernican Principle – the proposition that the Earth is not in a central or favoured position in the cosmos. Of course, it is well known that the movie’s principals, Robert Sungenis and Rick DeLano, are strict geocentrists who believe, for religious reasons of their own, that the Earth is absolutely static and located at the exact centre of the Universe. Strict geocentrism has been superseded for centuries – and there are clear modern refutations of the idea[3]. Strict geocentrism is also a far more extreme position than would necessarily follow if the Copernican Principle were to be violated. It is clear that The Principle movie is a Trojan Horse for strict geocentrism, even though DeLano in particular claims that the film contains no more than an examination of the Copernican Principle.
Chief amongst the recent cosmological measurements that geocentrists highlight in support of their case are certain anomalies in the Cosmic Microwave Background (CMB), the afterglow of the Big Bang. This article describes the science of the CMB, reviews the anomalies, and discusses what implications they have for cosmology in general and for the claims of the geocentrists in particular. We’ll also briefly review some other theories and observations that have the potential to challenge the Copernican Principle, and consider what implications they might have. In this article, I hope to provide a resource for thoughtful people who are unsure about the strength of the claims that the geocentrists make about modern cosmology, to help them to a better understanding of the science and what it actually implies.
The geocentrists approach to the issue is deeply unscientific, in the sense that they are committed a priori to the idea that the Earth is static in the exact centre of the Universe, and they excavate and use any piece of evidence that appears to support their case – even if it is fundamentally incompatible with some other argument that they have made elsewhere. Their belief is unshakeable in the face of any and all evidence to the contrary. What’s more, by misunderstanding or misrepresentation they often mangle the science, and they make far stronger claims than the evidence warrants, as we shall see.
The scientific approach is different: scientists attempt to build self-consistent models of the Universe. They try not to use arguments that are incompatible with others they use, or with observations, and they try to avoid making strong claims that are not warranted by the evidence. They also try to be open to the possibility that they might be wrong and that they need to follow where the evidence leads. As scientists are human beings, they don’t always live up to these ideals, but I believe that the community as a whole does succeed. In this article I will do my best to present a fair and balanced overview of the observations and what they could mean for our understanding of the Universe and for the geocentrists’ claims.
However, a fair description of the experimental observations and a cautious assessment of what they mean have resulted in arguments in this article that can be somewhat nuanced, complex and technical. I will try to keep the discussion as simple as I can but we will necessarily be led into more complex issues. If you are interested only in rhetorical one-liners in support of either side, this article is probably not for you. And whether or not you agree with my conclusions, I hope that you will learn something about the science that underpins modern cosmology.
2 What is the CMB?
2.1 The CMB predicted
I have described the Cosmic Microwave Background as the afterglow of the Big Bang, so let’s look at the evidence that supports that assertion.
There were two leading cosmological models in the middle part of the 20th century, of which the Big Bang idea was one[4]. Msgr Georges Lemaître had proposed the concept of the Big Bang in 1927 (although it wasn’t called that then) and it received substantial support in 1929 when Edwin Hubble discovered that the distances to galaxies were correlated with red-shift, meaning that the further away a galaxy is, the faster it is receding from us. Taken in conjunction with solutions developed by Alexander Friedmann to the Einstein field equations of General Relativity, which describe the evolution of a homogeneous and isotropic Universe, Hubble’s discovery was evidence for a Universe that was expanding everywhere. In such a Universe, you would observe galaxies receding faster the further away they are, regardless of your location in the Universe. If you look back in time in such a Universe then you arrive at a moment when all the mass and energy of the Universe is concentrated into a density that is so great that its behaviour cannot be predicted by currently known physics – this is the famous singularity that precedes the Big Bang.
The hypothesis that the Universe has a finite age and that it began with the Big Bang predicts that in its early stages it would have been immensely hot and would have contained a large quantity of radiant energy in the form of short wavelength photons. In the early Universe there was a period when the temperature was so high that electrons were unable to bind to protons to form neutral atoms. The photons of the early intense hot radiation were unable to travel very far before being scattered by the free, unbound electrons[5]. At some time later, the Universe cooled and expanded sufficiently so that the photons became able to stream freely across space (this event in cosmic history is known as “decoupling”, because photons and matter became decoupled at that time – subsequent measurements indicate that this occurred about 380,000 years after Big Bang). Cosmologists therefore predicted that the entire Universe is filled with these photons, which have been travelling freely across space since that time.
The theory also predicts that this radiation has a black body spectrum. The spectrum of a source is the intensity of the light as a function of wavelength; a “black body” absorbs all light incident on it and emits light with a very specific spectrum given by Planck’s law, where the peak of the spectrum (i.e. the wavelength with the highest intensity) is determined by the temperature of the body – in hotter bodies the spectrum is shifted to shorter wavelengths and in cooler bodies to longer wavelengths – see Figure 1. The Big Bang theory predicts that the photons of the early Universe would have an almost perfect black body spectrum.
The Big Bang theory also predicts that the primordial radiant energy will have been greatly cooled by the expansion of the Universe since the time of decoupling. This process maintains the black body spectral characteristics of the primordial radiation but shifts the spectrum to a much lower temperature. So, we should expect to find the Universe permeated by low temperature, long wavelength radiation possessing a black body spectrum.
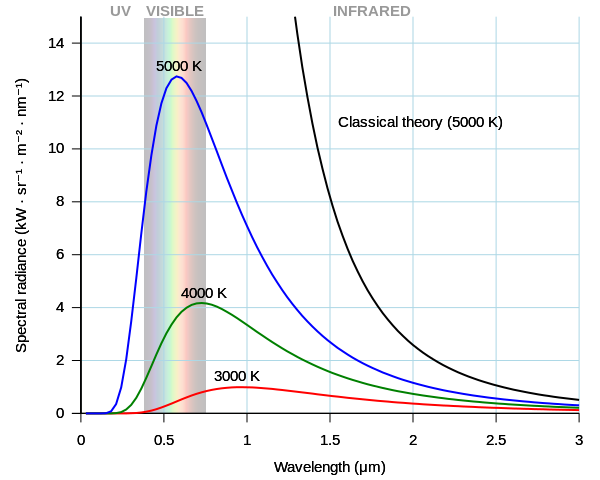
Fig 1. Three black body spectra at 3000K, 4000K and 5000K showing how the spectrum is shifted to longer wavelengths for cooler sources. The black curve shows the classical prediction for the spectrum of a black body, which does not match observations. In Planck’s theory the energy of radiation is quantised and the theory correctly predicts the black body spectrum. Source: Wikimedia Commons
These predictions were originally made by Ralph Alpher and Robert Hermann in 1948, forgotten, and then re-published by Robert Dicke and Yakov Zel’dovich in the early ‘60s.
2.2 Discovery of the CMB
In 1964, Arno Penzias and Robert Wilson of Bell Labs started work with a particular design of radiometer – a sort of telescope that can detect microwaves; microwave radiation is very long wavelength electromagnetic radiation. The radiometer was originally designed and built to communicate with satellites, but that function had become obsolete. They planned to use it for radio astronomy in the microwave part of the spectrum. Stars and other celestial bodies emit electromagnetic radiation right across the electromagnetic spectrum from short wavelength gamma rays to long wavelength microwaves, so astronomers are interested in all wavelengths.
Penzias and Wilson detected a signal that appeared to come from every part of the sky. At first they believed that it was an artefact of their instrument – the story goes that they spent days cleaning pigeon droppings off it in an attempt to get rid of what they originally thought was unwanted noise. However, it soon became clear that the signal was real and cosmological in origin.
The signal was very uniform across the sky – it appeared to be the same intensity in whatever direction they looked and subsequent measurements at different wavelengths confirmed that it had a perfect black body spectrum. In fact, this radiation “has the most perfect black body spectrum ever measured”.
It was soon clear that they had detected the radiation predicted by Alpher and Hermann, and Penzias and Wilson were awarded the Nobel Prize for physics in 1978. The radiation became known as the Cosmic Microwave Background (CMB) and its characteristics were about to reveal a great deal about the early Universe.

Fig 2. The most perfect black body spectrum ever measured. The spectrum of the CMB measured by the COBE satellite. The data points are indistinguishable from theory and the error bars of the measurement are too small to plot. Source: Wikimedia Commons
2.3 Characteristics
The temperature of the CMB is 2.73K, or just 2.73° above absolute zero. As the temperature of the early Universe dropped to below 3000K, there were not enough sufficiently energetic photons to prevent the electrons from binding, and the free electrons were captured by protons to form hydrogen atoms (each neutral hydrogen atom consists of one proton, the nucleus, and one bound electron), which allowed the primordial radiation to propagate freely. This process is called “decoupling” or “last scattering”. The temperature of the CMB at the time of decoupling was therefore about 3000K, and the Universe has expanded by a factor of about 1089 since that time (The theory indicates that the temperature is inversely proportional to the scale factor, which is a measure of how much the Universe has expanded).
The temperature of the CMB is extremely isotropic – in other words, it looks the same in all directions. It is very important to understand just how uniform the CMB is. The fact that the CMB is so isotropic is good evidence for the Cosmological Principle that states that the Universe is homogeneous and isotropic on large scales.
However, the temperature of the CMB is not completely uniform and there are random fluctuations or variations of the order of one part in 100,000, or about 18μK (18 millionths of a degree). These fluctuations are known as anisotropies in the CMB – departures from perfect isotropy (isotropy means that something looks the same in all directions). If the CMB is ancient radiation that arises from the early Universe, then how do these anisotropies arise and what can they tell us about the cosmos?
The basic pattern of anisotropies is predicted to arise from primordial quantum fluctuations in the very early Universe[6]. Quantum theory predicts that the early Universe would not have been completely uniform but that there would have been random variations in density. Such variations in density would have survived through the early epoch of the Universe prior to decoupling and in fact would have been modified by the behaviour of the early Universe in a way that provides us with information about the make-up and behaviour of energy and matter prior to decoupling. Those parts of the CMB that arose from regions of higher density would be cooler, compared to parts of the CMB that arose from less dense regions[7]. These are called primary anisotropies.
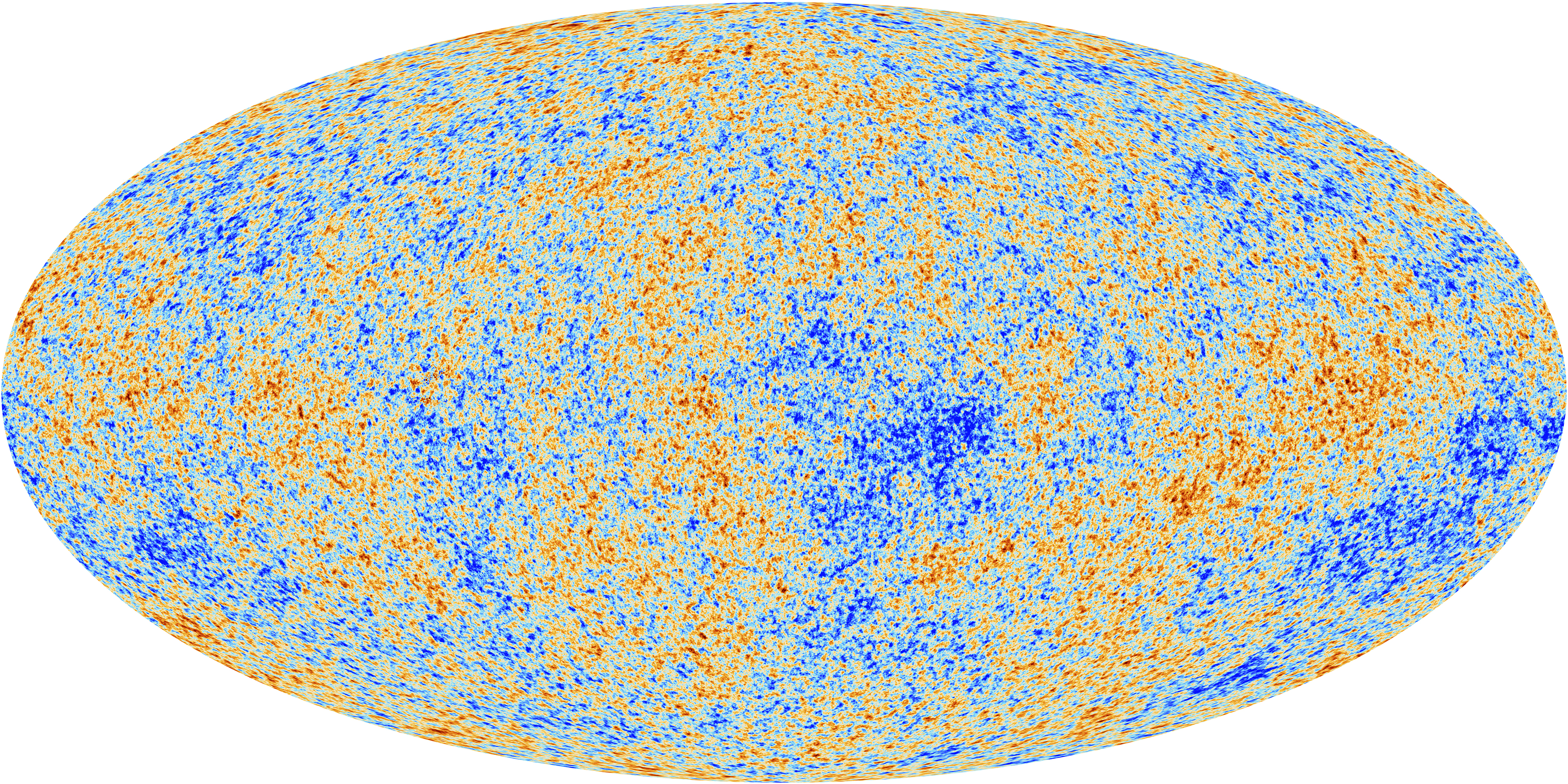
Fig 3. A cleaned full sky map of the Cosmic Microwave Background from the Planck10 mission showing temperature anisotropies – hotter regions are coloured red, and cooler regions blue. Source: ESA; Planck Collaboration
There are also anisotropies in the CMB that arise after decoupling – processes that affect the temperature and paths of the CMB photons as they stream across the Universe. An example of this is known as the Integrated Sachs-Wolfe effect: the CMB radiation temperature is gravitationally affected by the photons’ passage through or near to massive structures such as galaxies or galaxy clusters. These are called secondary or late anisotropies.
Turning back to the primary CMB anisotropies, the statistics of the fluctuations carry a great deal of information about the processes that took place prior to decoupling. As you will remember, the Universe was opaque to radiation prior to decoupling and so it seems surprising that we can see what was taking place in the Universe then. However the processes that occurred imprinted certain patterns of temperature fluctuation on the CMB at the time of decoupling and so allow us to look through what seems at first sight, an opaque and impenetrable curtain.
2.4 The statistics of the CMB
We have seen that the temperature fluctuations in the CMB are random, and it is the statistics of those random fluctuations that allow us to probe the Universe before and after decoupling. The quantum theory of the Big Bang predicts that the primordial fluctuations were Gaussian and nearly scale invariant. The statistics of the CMB temperature fluctuations reflect this Gaussian and near scale invariance. So what do Gaussianity and scale invariance mean? Gaussian statistics means that the temperature fluctuations in the CMB should follow a Gaussian or bell-curve distribution as in Fig 4. The bell curve is centred on the mean temperature of 2.73K and the probability of measuring a particular temperature at any point on the sky is given by the curve. The percentages show how much of the distribution falls within that segment of the curve.
The original quantum fluctuations are also predicted by quantum theory to have been nearly scale invariant – that is the density fluctuations in the primordial plasma would have had almost the same amplitude at all scales. (Subsequent physical effects that occurred after Big Bang and before decoupling have intensified the fluctuations at some scales while suppressing the fluctuations at other scales.) A modest deviation from scale invariance in the primordial fluctuations is evidence for cosmic inflation (see Section 3 below on the Standard Model).
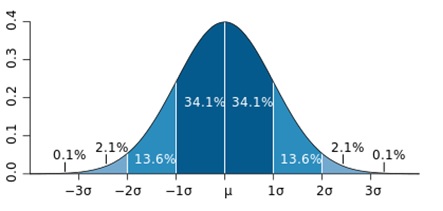
Fig 4. A Gaussian distribution. In the case of the CMB the vertical axis is probability of that temperature and the horizontal axis is temperature in degrees K where μ is 2.73K and 1σ is ~18μK. Source: Wikimedia Commons
The distribution of the temperature fluctuations in space, across the sky, can be characterised by decomposing the whole sky temperature map, as in Fig 3, into spherical harmonics also known as spherical multipoles. We do this in order to see how the amplitude of the temperature fluctuations varies according to their scale. This is analogous to the well-known decomposition of a one-dimensional signal into discrete Fourier components[8]. The full anisotropy map is made up of a series of discrete spherical multipoles, where these multipoles are characterised by increasing numbers of lobes equal in number to integer powers of two (2, 4, 8, 16 etc.). These multipoles start at l=0 (which is the basic temperature of the CMB at 2.73K), and continue through l=1 (the dipole, in which there are two lobes on the sky, where one lobe is hotter than the other), l=2 (the quadrupole with four lobes interleaved,), l=3 (the octopole with eight lobes interleaved), and so on indefinitely. In principle there are an infinite number of multipoles making up the CMB anisotropy. If we add together all the multipoles we would reconstruct the original full sky map as in Figure 3.
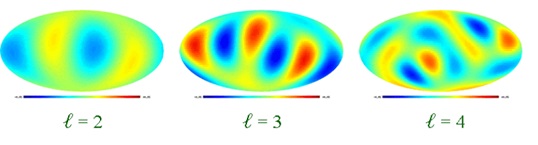
Fig 5. The quadrupole, octopole and hexadecapole of the CMB where the extreme colours represent ±50μK. Source: NASA WMAP 3 Year ILC Map
I’ve already mentioned that the primordial Gaussian, nearly scale invariant fluctuations are modified by events that took place in the Universe in the epoch before decoupling, so we’ll look now at what those events were. For 380,000 years after Big Bang, the Universe consisted of a plasma of baryons (protons and neutrons) and radiation, the so-called photon-baryon plasma. Matter was gravitationally attracted to regions of slight over-density, whereas the interaction of radiant energy with matter in those regions created a pressure that tried to push the matter apart. The resulting tension caused density waves, similar to sound waves with which we are all familiar. The detailed theory of this epoch of the Universe[9] predicts that these “sound waves” (known as Baryon Acoustic Oscillations or BAO) intensified regions of over- and under-density in the Universe. The speed of sound in the baryon-photon fluid was about 0.58x the speed of light. These oscillations occurred at a wide range of scales because of the scale invariance of the original fluctuations in density that gave rise to them. The oscillations occurred for a finite time from the Big Bang to decoupling, about 380,000 years later, after which the pressure from the photons ceased when the electrons combined with protons to form neutral atoms and the photons were able to propagate freely across space. At that time the variations in density in the fluid were imprinted on the temperature of the photons – hotter where the photons arose from regions of lower density and cooler from regions of higher density7, and these photons are the ones that we detect now in the CMB.
If we consider which scale had the greatest variation in density at the time that the density fluctuations were imprinted on the CMB then we can see that this is the scale that accommodates a single compression cycle in the time available. At other scales, the oscillations would have been part way through a compression-rarefaction cycle at the time of decoupling and therefore would have had less amplitude. Theorists therefore predict that the BAO structure would have maximum amplitude at a scale at decoupling known as the sound horizon – the distance sound waves could travel across the Universe in the 380,000 years between Big Bang and decoupling. After decoupling, the photons are free to stream away across the Universe carrying the imprint of the acoustic oscillations with them. These acoustic oscillations, the ringing of the early Universe, can be seen in the statistics of the CMB anisotropies. Taking the expansion of the Universe into account, theorists predicted that the first peak in the anisotropy power spectrum (the plot that shows the intensity of each multipole versus multipole number) would occur at about multipole l=220, which subtends approximately 1° on the sky. The exact location of the measured peak and the angle that the peak multipole measures on the sky gives us information about the geometry of the Universe, indicating that it is flat or nearly so.
The CMB anisotropies contain much more information. For example, the second peak in the anisotropy spectrum carries information about complete cycles of compression and rarefaction in the acoustic oscillations and the ratio of the amplitudes of the first and second peaks tells us about the density of matter and the ratio of matter to radiation.
In addition to these primordial anisotropies, the CMB is also affected by its propagation across the Universe for 13.8 billion years, and carries other information about the Universe structure in anisotropies that have been created since decoupling – the so-called late or secondary anisotropies, which include gravitational red and blue shifting (the Integrated Sachs-Wolfe effect), increased photon energy caused by scattering off high energy electrons (the Sunyaev- Zel’dovich effect) and gravitational polarisation. These effects also contribute to our cosmological knowledge.
The fact that the CMB is the oldest light in the Universe and is very uniform across the sky is interesting in itself, but the very faint anisotropies in the CMB, which open a window to the primordial Universe, are the key to cosmologists’ interest in the subject.
2.5 Probing the CMB
Because the CMB consists of such ancient light and because it carries so much information about the early Universe, there have been many experiments to measure it. The most well-known are the three satellite experiments, COBE[10] (1989 – 1996), WMAP[11] (2003 – 2010) and Planck[12] (reporting at the time of writing). These satellites measured the CMB anisotropies across the entire sky. Each satellite was able to measure the CMB with greater precision and in finer detail than its predecessor.
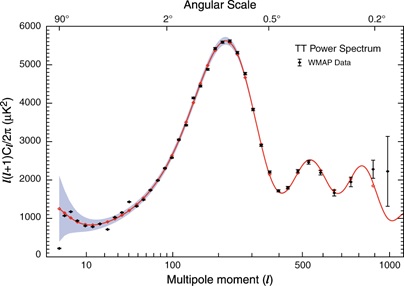
Fig 6. The three-year WMAP CMB power spectrum. The vertical axis represents the amount of anisotropy and the horizontal axis represents the scale, with large scale modes towards the left and fine scale modes to the right. The theoretical prediction of the standard ΛCDM model is the red curve and the experimental data are the black points with error bars. Source NASA/WMAP Science Team
COBE confirmed the very high homogeneity, isotropy and Gaussianity of the full sky CMB, and was the first mission to detect the predicted anisotropies. However, its resolution was insufficient to measure the first acoustic peak predicted at l = 220. The first acoustic peak was first measured by two high altitude balloon experiments (BOOMERanG[13] and Maxima[14]), which measured the anisotropies in the CMB beyond l=220 over a small area of the sky.
WMAP gathered data over nine years and confirmed that the first acoustic peak was at l ≈ 220 as predicted. Many details of the WMAP detailed measurements were consistent with the Standard Model of cosmology (the Big Bang, inflation, dark energy, cold dark matter model).
The European Space Agency operated the Planck satellite between 2009 and 2013. The first year results were published in 2013 with more results, and in particular the polarisation data, expected in 2014. With a greater resolution than WMAP and higher precision radiometers, Planck was able to measure the CMB anisotropy out to l = 2500 which is equivalent to 0.07° or about 4 arcmin scale on the sky. Planck provided greater precision than WMAP but the basic conclusion it reached is the same – the CMB data fits the standard ΛCDM model (see Section 3 below) very closely indeed. The overview paper states[15]: “Scientific results include robust support for the standard, six parameter LCDM model of cosmology and improved measurements for the parameters that define this model, including a highly significant deviation from scale invariance of the primordial power spectrum.” The modest but significant deviation from scale invariance is evidence for cosmic inflation.
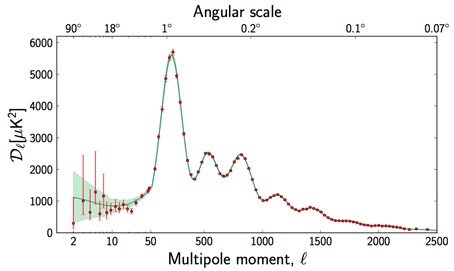
Fig 7. The Planck CMB power spectrum. The best fit ΛCDM model is the green curve and the shaded area shows cosmic variance (the statistical variation expected in the best fit model from one observational location in the Universe to another). The data points and error bars are red. The measured CMB matches the six-parameter ΛCDM model closely across all seven peaks. Source: ESA and the Planck Collaboration
The paper also points out that the Planck data is highly consistent with other observational data such as Big Bang Nucleosynthesis[16] and states “The predictions of the baryon density from these two methods involve all of the known forces of nature and this highly non-trivial consistency provides strong evidence for the universality of those laws.”
So, a key finding of these measurements of the CMB by the WMAP and Planck satellites, along with other CMB measurements made from high altitude balloons and ground based microwave radiometers is that the CMB anisotropies fit the Standard Model of cosmology to an extremely high degree of accuracy.
3 Standard Model of the Universe
In this section we’ll look at what is known as the Standard Model or Concordance Model of cosmology. There is a very powerful consensus that the Standard Model is a good approximation to the observable universe, although most if not all cosmologists would agree that it is not correct in every detail and that it needs to be refined.
3.1 The Copernican and Cosmological Principles
The Standard Model starts from the assumption that what we observe of the Universe is typical of what would be observed from any vantage point – this assumption is based on the Copernican Principle, and its more stringent version, the Cosmological Principle.
The Copernican Principle proposes that the Earth is not at a central or otherwise special or privileged place in the cosmos. Galileo observed other solar planets with their moons orbiting the Sun and concluded that the Earth was also a planet orbiting the Sun. In the 20th Century, astronomers looked out to the distant Universe through land-based and satellite telescopes such as the Hubble Space Telescope and observed a vast quantity of galaxies. We came to understand that the Sun is just one of a huge number of entirely unremarkable stars that make up the Milky Way Galaxy, which itself is just one of a huge number of spiral galaxies in the observable Universe. Current estimates suggest that there are about 100 billion stars in the Milky Way and between 100 billion and a trillion galaxies in the observable Universe. Our observations support the Copernican Principle.
The Cosmological Principle goes further and makes a more precise and stringent claim: the Cosmological Principle states that the Universe is homogeneous (it has a similar structure everywhere) and isotropic (it looks the same in all directions no matter from where you observe it). The Standard Model assumes homogeneity and isotropy on the very largest scales – obviously on the scales of galaxy clusters and the intervening voids the Universe is not homogeneous – there is clumping of matter into stars, galaxies and galaxy clusters that violates homogeneity and isotropy on those scales and the Standard Model actually explains why that is. The very high uniformity of the CMB is evidence for the Cosmological Principle, and the Standard Model largely accommodates and predicts the departures from strict isotropy that are observed.
3.2 General relativity and the FLRW metric
The best current theory of gravity is Einstein’s Theory of General Relativity (GR). It is based on the Einstein field equations first published in 1915 that describe how mass and energy in the Universe distorts space-time and how the distortion of space-time affects the motion of matter and the propagation of radiation. In order to use GR to describe phenomena and make predictions about the Universe, scientists must find solutions to the field equations for various specific circumstances. The solution that is the basis for the Standard Model of cosmology is the Friedmann-Lemaître-Robertson-Walker metric that describes an expanding or contracting homogeneous and isotropic Universe filled with a perfect fluid. There are many other cosmological solutions to the Einstein field equations, but many of these make unphysical predictions– in other words predictions that do not fit what we observe. The evidence gathered so far is that the Universe as a whole is well described by the FLRW metric but there is also some speculation that other solutions that describe modest departures from homogeneity correspond better to regions of the observable Universe – we’ll discuss these ideas later.
3.3 Inflation
There were two cosmological problems inherent in the Big Bang model that remained unsolved up until the mid-1980s[17]:
- The Horizon problem: there are parts of the observable Universe that are too far apart to ever have been in direct causal contact and yet the Universe is very homogeneous
- The Flatness problem: the geometry of the Universe is measured to be flat or nearly flat, which would require extreme fine tuning early on
To resolve these issues, Alan Guth proposed the theory of inflation[18] that states that the Universe went through a period of extremely rapid expansion by at least a factor of 1026 in 10‑33 seconds just after Big Bang. This would allow parts of the Universe, which are now very far apart, to have been in causal contact before the period of inflation; and it would provide a mechanism for creating a flat Universe without fine tuning.
There are several different models for the inflationary process and for the field that is thought to have driven it. The favoured model makes a number of predictions about things that depend on inflation, which we can observe in the current Universe, and those predictions have been broadly fulfilled. This gives significant credence to the concept.
For example, inflation predicts that the anisotropies in the CMB and the large scale structure of galaxies originate from quantum perturbations in the inflation field. Quantum theory is the basis for determining the statistics of the perturbations. The CMB was predicted to be highly uniform, with small anisotropies that correspond to inflationary quantum perturbations. The predicted anisotropies are Gaussian and almost, but not quite scale invariant, as we saw in Sec 2.4 above. Measurements from COBE through WMAP and Planck, as well as large scale surveys of galaxies are in good agreement with the inflation predictions. Other predictions of inflation such as a flat geometry for the Universe and perturbations that are adiabatic[19] are also well supported by the data.
So, although at first sight inflation seems to be a rather arbitrary hypothesis, spirited up to solve a few cosmological problems, the theory does make very specific predictions that are closely confirmed by a range of observations.
3.4 Dark Matter
It has been known since the 1930s that the orbital characteristics of the Milky Way and the orbital velocities of galaxy clusters are such that much more mass is present than can be seen. The suggestion that there is an exotic form of matter, which interacts gravitationally but does not interact through other physical forces and so cannot be seen, was made first by Fritz Zwicky in 1937[20].
In the late 1970’s and early 1980s, Vera Rubin and colleagues[21] made precise measurements of the speed of stars in other galaxies versus their radial distance from the centre of the galaxy (known as the rotation curve). They found that in spiral galaxies the speed of stars was approximately independent of distance from the centre of the galaxy (this is known as a flat rotation curve), whereas the distribution of mass in the galaxy determined by the luminosity of stars predicted that the speed of stars should reduce further from the galactic centre (see Fig 8). The observation can be explained if we postulate a large mass of non-luminous matter in the galaxy extending well beyond the central part of the galaxy where the majority of the stars are found[22]. The missing mass appears to be an exotic form of matter, called dark matter, which interacts only gravitationally with ordinary matter and radiation, and which has so far not been identified in the lab.

Fig 8. Predicted (A) without dark matter and observed (B) rotation curves for a typical spiral galaxy. Source Wikimedia Commons
More recently, measurements of gravitational lensing (the bending of light when passing near to very massive objects as predicted by General Relativity) of background objects by foreground galaxies is consistent with the presence of dark matter.
One observation is particularly compelling: the Bullet Cluster of galaxies consists of two clusters of galaxies that are part way through colliding. Gravitational lensing and conventional astronomy in the visible and X-ray spectra show that the dark matter and most of the ordinary matter in the form of hot gas have become separated in space. Hot gas within the clusters interacts electromagnetically as well as gravitationally and has been slowed down by the collision – dark matter is not affected electromagnetically and so the dark matter of the two clusters appears to have passed unaffected one through the other.
The CMB itself is affected by the presence of dark matter. The detailed models, which determine the evolution of the initial perturbations in the Universe, and which eventually give rise to the anisotropies in the CMB as well as the distribution of both ordinary and dark matter, are consistent with the Standard Model, including the theory of Baryon Acoustic Oscillations. The statistics of the CMB are consistent with the dark matter hypothesis.
Alternative explanations for the observations have been proposed, such as modifications to Newtonian gravity. Although some of these alternative hypotheses match some of the observations well, none is as consistent with the full range of observations as the Standard Model incorporating dark matter.
The particle that comprises dark matter has not been identified. Some candidates have been eliminated by experiment, although there are many candidates for which there are yet to be tests. Many different sorts of observation provide evidence for the existence of some form of dark matter, but it is true to say that physicists will not be entirely comfortable on this score until either its constituent exotic particle is detected directly, or physicists come up with some alternative physics that explains the observations at least as well as the current explanations.
3.5 Dark energy
A supernova is the huge explosion of a star, which in some cases can briefly outshine the luminosity of an entire galaxy. Supernovae are thought to be caused by a number of different mechanisms that are classified by the spectral characteristics of the light emitted and by the details of the light curve (the light curve is how the intensity of light emitted by the supernova grows and fades over time). One particular sort of supernova, known as Type 1a[23], has extremely uniform characteristics – Type 1a supernovae all behave the same and so they are very useful as standard candles to measure distances in the Universe.
Two exciting and unexpected papers were published in 1998. Adam Riess et al[24] and Saul Perlmutter et al[25] investigated the distant Universe using Type 1a supernovae and discovered that the expansion of the Universe is currently accelerating by comparing the brightness of these supernovae to their redshift. This was unexpected – it had been known since 1929 when Edwin Hubble made his observations of redshift that the Universe is expanding, but the cosmologists expected that the expansion would be slowing down under the gravitational influence of the matter in the Universe.
Furthermore, if the Universe is flat (i.e. the curvature of space-time is zero), the mass-energy density in the Universe must be a certain critical level. WMAP confirmed that the Universe geometry is flat or near to flat. The mass density of ordinary and dark matter is just 30% of the critical level according to observations on a large scale of the number density and mass of galaxies[26]. Further evidence for the existence of dark energy comes from the CMB itself[27].
If there is a kind of energy in the Universe accelerating the expansion, then the density of that energy must be added to the density of ordinary and dark matter in determining the total density of the universe. Therefore, cosmologists have proposed the existence of dark energy, which explains two observations:
- The total mass energy density of ordinary matter, dark matter and the proposed dark energy is close to the critical density which explains why the curvature of space-time is near or equal to zero
- The dark energy causes a pressure which explains the observed acceleration of the expansion of the Universe.
The actual nature of dark energy is currently a mystery. The FLRW solution to the Einstein field equations (see Section 3.2 above) has a term called a cosmological constant, which is represented by the Greek capital letter lambda (Λ) in the Friedmann solution. If the cosmological constant is non-zero then it could have a value that would explain the accelerating expansion and the critical density. Such a constant is allowed in GR and would be constant in time and space and could represent the energy of the vacuum. Other ideas include a scalar field called quintessence, which can vary in time and space. Current measurements of the Universe indicate that the density of dark energy does not change as the Universe expands, which is consistent with dark energy being a cosmological constant.
3.6 The ΛCDM model
So, taking all these interlocking observations into account, and many more that we don’t have the space to explore, the current Standard Model of cosmology is the ΛCDM model, where Λ represents dark energy and CDM stands for Cold Dark Matter.
The ΛCDM model is based on the Big Bang with inflation and describes a homogeneous isotropic expanding Universe that has flat geometry and in which the expansion is currently accelerating.
It might seem strange that we are familiar with and have characterised in detail merely 5% of the matter and energy that makes up the Universe – some 25% in our best model is dark matter and 70% is dark energy, and frankly we don’t know at the moment what dark matter and dark energy are. Some people, particularly those who have a bone to pick with modern science, criticise modern cosmology for “inventing” dark matter and dark energy. They use every trick of rhetoric to suggest that these concepts are arbitrary and ill-evidenced, and that modern cosmologists are either incompetent, engaged in a massive conspiracy or both.
The fact is that physicists have not arrived at the ΛCDM model lightly. As we have seen, there are extremely good reasons for proposing the existence of the Big Bang, inflation, dark matter and dark energy. One of the key pieces of evidence is the CMB itself. Its uniformity to one part in 100,000 supports the concept of a homogeneous and isotropic Universe. ΛCDM has only six free parameters and yet it predicts the details of the power spectrum of the CMB, to a quite remarkable precision out to l=10,000[28]. Further parameters can be derived from the best-fit six-parameter model, which are consistent with observations. As well as measurements of the CMB, there are many independent observations of the Universe, such as large scale surveys of galaxies, gravitational lensing, measurements of the Hubble constant and so on, which either are direct evidence in support of the model or are consistent with it.
Although the Standard Model fits the observations very well, few, if any, physicists would claim that it is accurate in all respects. Nor is the scientific community complacent. A great deal of work is underway to refine the model and to resolve discrepancies. Collaborative physics projects are underway or planned to search for the origin and nature of dark matter – the need to understand its nature is widely acknowledged by the physics community. Theoreticians are considering and publishing alternative cosmological models that include ideas based on modified gravity, and potential departures from homogeneity and isotropy are under active consideration.
The New Geocentrists would have you believe that ΛCDM, indeed all of modern cosmology, is a theory in crisis and that the entire edifice is about to collapse, clearing a building site for a geocentric temple. The reality is different – astronomical and astrophysical observations over the last two decades have strengthened the evidence for the Standard Model or something very like it. There is no conspiracy of silence – anomalies and other pieces of evidence challenging the Standard Model, such as they are, are all detected, identified, published and discussed openly by the physics community without whom the new geocentrists would not be aware that the CMB exists, much less that there are unexplained anomalies associated with it.
Is it possible that ΛCDM is entirely wrong and that cosmologists, astrophysicists and theoretical physicists have been barking up the wrong tree for decades? Of course it is possible. There is no absolute proof in science and it is possible that some future observation or combination of observations will force a reassessment of the fundamentals. However, given the complex interlocking empirical evidence it is unlikely that ΛCDM is entirely wrong; and even if it is, that doesn’t imply that geocentrism or anything like it is correct. The fallacy of False Dichotomy, “you are wrong so I must be right”, is one of which the geocentrists are frequently and unashamedly guilty. With that in mind, it’s time now to look at the anomalies in the CMB that don’t appear to fit the Standard Model.
4 Anomalies in the CMB
So, what are the anomalies in the CMB? The inflation-driven Big Bang ΛCDM model makes certain predictions about the statistics of the random fluctuations in the temperature of the CMB. We have seen that measurements show that those predictions are met to a remarkable degree – see Fig 7. Other predictions, such as the Gaussian distribution of temperature are also closely met[29]. Nevertheless, detailed analysis of the statistics and structure of the CMB anisotropy reveals features that are not predicted under the Standard Model and it is these anomalies that we are going to review now.
4.1 What are the anomalies?
The anomalies were hinted at by the COBE satellite data, but were first fully identified by WMAP and have since been confirmed by the Planck satellite. Let’s look at the most important ones:
- You will remember that the anisotropy power spectrum shows how much the temperature of the CMB varies as a function of the scale or angular size of the fluctuations (see Figs 6 and 7). Compared to the predictions of the model, the CMB anisotropy lacks power at the largest scales. The quadrupole (with four lobes, two hot and two cold) and to a lesser extent the octopole (with eight lobes) show less difference in temperature between hot and cold lobes than predicted[30].
- According to the Standard Model, the alignment between the lobes of various multipoles of the CMB anisotropy should be random. WMAP found a significant and anomalous alignment between quadrupole and octopole. Moreover these were approximately aligned with the autumnal equinox and the dipole. The equinoxes are two points on the celestial sphere where the plane passing through the Earth’s equator and the plane of Earth’s orbit round the Sun – the ecliptic plane – intersect. The CMB dipole means that the CMB is hotter in one direction than the opposite direction by about one part in a thousand, which is conventionally explained by a Doppler shift of photon energies in the CMB caused by the motion of the solar system through the Universe with respect to the rest frame of the CMB[31]. Planck found the same alignments at a somewhat lower significance. See section 4.2 for a detailed description of these alignments, which are a major plank in the geocentrists’ attack aimed at discrediting the Copernican and Cosmological Principles, and proving geocentrism.
- The Standard Model predicts that not only should the temperature of the CMB be uniform wherever we look, which, as we have seen, it is to a remarkable degree, but that the anisotropies should be the same wherever we look. The Planck CMB data confirms earlier measurements that indicate that there is more variation in the temperature in one hemisphere versus the opposing hemisphere[32], as well as other differences in the statistics in hemispheres divided by the galactic plane (the plane of the Milky Way galaxy) and more significantly in hemispheres divided by the ecliptic plane (the plane of Earth’s orbit round the Sun).
- The data shows that there is a significant degree of anisotropy power asymmetry, which varies by direction depending on the scale of the anisotropies[33]. In other words there is more temperature variation in one part of the sky than another and the direction of the greatest variation is different for different scales. The amount of this asymmetry is not greater than predicted by the theory, but there is significant clustering of the direction of the asymmetry around a preferred direction that is anomalous.
- Planck confirms earlier findings that odd multipoles have somewhat high power compared to even multipoles[34]
- There is a statistically anomalous Cold Spot in the CMB sky[35]. The Cold Spot subtends about 5° on the sky. It has been extensively studied[36] and physicists have made various suggestions for its cause.
4.2 The Anomalous Alignments
Since the geocentrists focus particularly on the CMB alignments that are not predicted by the Standard Model, let’s take a deeper look at these in preparation for a fuller discussion of their claims.
The alignment between the CMB dipole and the equinox has been known for over 30 years. The magnitude and direction of the dipole have been refined by many probes of the CMB including the satellites COBE, WMAP and Planck. The measured values for the direction and amplitude of the CMB dipole have remained the same for a decade or more. The direction of the CMB dipole vector is 14.1° from the autumnal equinox (The way the geocentrists describe these alignments you’d think that they are exact). No sensible person suggests that this alignment on its own is remarkable.
Not only are the quadrupole and octopole approximately aligned to one another but they are also approximately aligned with the equinox and the dipole. 9-year WMAP data showed a highly significant alignment of just 3° between quadrupole and octopole[37]. The more precise Planck satellite data found a less exact alignment – between 9° and 12.3° which reduces to 7.6° to 8.3° when certain contributions to the quadrupole from the solar system velocity with respect to the CMB rest frame are subtracted. The significance of the alignment of quadrupole and octopole lies between 97% and 99% (in other words there is only a 1% to 3% chance that it occurs by chance).
Using the data from Table 18 of ref [29], which shows the direction in galactic co-ordinates of quadrupole and octopole as measured by Planck for a number of different component separation schemes (which are algorithms used to clean the CMB maps of foreground contamination, which is microwave energy that arises from sources that are not part of the CMB), I have calculated the measured angles between various features. The angles between quadrupole, octopole, dipole, equinox and ecliptic plane for the KQ corrected SMICA component separation scheme are:
- The quadrupole to the equinox is 23.1°
- The octopole to the equinox is 17.6°
- The quadrupole to the dipole is 28.5°
- The quadrupole to the octopole is 7.7°
- The dipole to the equinox is well known and is 14.1°
- The dipole to the ecliptic plane is 11.1°
- The quadrupole to the ecliptic plane is 16.0°
- The octopole to the ecliptic plane is 8.6°
So the alignment of these CMB features with themselves, with the kinematic dipole caused by the motion of the solar system, and with the equinox, is far from exact and lies within a 30° cone. Nevertheless this alignment of a number of nominally independent directions is unexpected and the probability that all four align to this degree randomly is only about 0.3%.
The consensus is that alignment of the equinox, dipole, quadrupole and octopole at about a 3σ level is significantly present in the data (i.e. only 0.3% probability that they all occur by chance). The probability that any two align systematically given that the other two align with them and with each other by chance is much higher, of course, at between 2.5% and 5% as Copi et al have shown in their review of the low multipole alignments as measured by Years 7 and 9 data from WMAP and Year 1 data from Planck[38]. They demonstrate that the alignments of the quadrupole and octopole are significant at the levels we have discussed, but that their alignment with the dipole may well be a statistical accident. They assess the alignment of the quadrupole and octopole with respect to the ecliptic rather than the equinox itself, and conclude that although solar neighbourhood explanations for the alignment are plausible, no specific explanation of this kind has been found. They state:
“The statistics offer only weak, and even confusing, guidance as to what is correlation and what, if anything, is causation. … Unfortunately, the fact that the dipole direction simply happens to be just off the Ecliptic plane, which passes about 30 degrees from the Galactic poles, makes establishing the priority of one correlation over another difficult just on the basis of statistics of CMB temperature data. Some, or all, of these correlations are presumably accidental”
They conclude:
“We think it is preferable to acknowledge that the existence of anomalies seen in the WMAP and Planck maps at large angular scales may point to residual contamination in the data or to interesting new fundamental physics.”
A contrary view was expressed by Bennett et al in one of the papers published by the WMAP team[39]. They suggest that the significance of the anomalies as cosmic phenomena is less than is widely claimed in the literature, owing to the influence of what is called a posteriori choice of statistics. In other words in a complex data set (and the CMB data set is very complex), there are bound to be some coincidences and we are focusing on the anomalies we have found, to the exclusion of the vast quantity of non-anomalous data. Some anomalies are expected to exist in any complex dataset purely statistically. It is worth quoting extensively from the abstract:
“A simple six-parameter ΛCDM model provides a successful fit to WMAP data… While there is widespread agreement as to the overall success of the six-parameter ΛCDM model, various “anomalies” have been reported relative to that model… In most cases we find that claimed anomalies depend on posterior selection of some aspect or subset of the data… We conclude that there is no compelling evidence for deviations from the ΛCDM model, which is generally an acceptable statistical fit to WMAP and other cosmological data.”
See also [40] for an earlier paper by Copi et al published at about the same time, where they argue that the anomalies are present and significant.
Since Bennett et al reported in 2010, the Planck team have published the data that we discussed above. The consensus of the scientific community now is to adopt the working assumption that the anomalies are statistically significant and are not merely accidents that have been made prominent by an a posteriori choice of statistics. The consensus position is to accept that the ΛCDM model is the best fit to the known data, but that the anomalies might be signals for new physics that would lead to some modification or refinement of the model. It seems to me that this is a prudent approach, which avoids the pitfall of potentially missing some important new physics. There is nothing to lose by tentatively treating the anomalies as significant, but potentially much to lose by treating them as statistical flukes.
4.3 Potential Explanations for the Anomalies
There have been a substantial number of ideas advanced to explain the anomalies:
- An artefact of our focus on anomalies and the a posteriori choice of statistics as described in the previous section
- Instrument systematics: there is a possibility that something in the design or operation of the satellite radiometers gives rise to data artefacts that cause the apparent alignment. This is extremely unlikely now that Planck, which uses a very different instrument design from WMAP, finds the same anomalies albeit with somewhat reduced significance
- Data analysis systematics: this covers a wide range of possible errors – the data analysis includes the various filters applied to reconstruct the data, the cleaning of the raw data to remove foreground, and to correct for systematic late time effects on the primordial CMB such as the Integrated Sachs-Wolfe effect and gravitational lensing, which can lead to the sort of anomalies we see. It is fair to say that people have looked hard for systematic data analysis artefacts to explain the anomalies and have failed to find them and, again, since different experimental designs find the same anomalies, it seems unlikely that the explanation will be found here (although Copi cautions that the First Year Planck data calibrates against the WMAP dipole)
- Foreground contamination: in attempting to measure the CMB, there is a possibility of foreground sources of microwaves contaminating the data. Such sources can lie within the solar system, or be galactic or extra-galactic. A considerable amount of foreground contamination is removed in reconstructing the cleaned full sky CMB maps. The foreground contamination includes point sources (i.e. astronomical objects such as stars and galaxies), synchrotron emission, thermal Bremsstrahlung, thermal dust emission, and so on. (We don’t need detailed descriptions of these potential sources of foreground – we just need to know that these are all physical processes that can contaminate the measurement of the primordial radiation.) It is possible that the anomalies are due to imperfect cleaning of foreground contamination, but that is less likely now since the Planck publications find the same anomalies, in spite of the fact that Planck and WMAP use different component separation algorithms (and Planck actually makes use of four different algorithms). However, foreground contamination as a source of at least some of the anomalies remains a possibility
- Secondary or late-time anisotropies: the CMB light is known to be affected by its 13.8 billion year passage through the Universe by a number of effects, which include scattering from electrons, gravitational lensing and gravitational red-shift. These effects tell us a great deal about the Universe, but must be corrected for in order to get the statistics of the CMB anisotropies as they were at the time of decoupling. It is possible that some as yet unidentified but systematic late-time anisotropies remain in the cleaned data that can explain the anomalies, but there is currently no compelling suggestion for what they might be
- New physics. It is possible that the anomalies arise from real processes in the Universe, which cause some departure from strict homogeneity and isotropy on a large scale. Amongst other explanations, astrophysicists and theoretical physicists are investigating potential sources of anisotropy in the early Universe that are not currently part of the Standard Model, such as primordial texture and topological defects. Some metrics that are not flat, isotropic and homogeneous, when overlaid on the FLRW metric, explain the alignments to some degree[41]. It is possible that primordial magnetic fields on the scale of the current horizon can give rise to a preferred angular direction in the CMB. It is also possible that the departure from strict anisotropy indicated by the alignments can be accommodated by the Standard Model as a very large scale primordial anisotropy falling within the observable horizon[42]. So far, although there have been many physical explanations proposed for the alignments, no one appears more compelling than others.
The Planck team has so far not released any CMB polarisation data, which is due in late 2014. The correlation or lack of it, between temperature and polarisation anisotropies can constrain explanations and help in the search for a cause and so the Planck polarisation data is eagerly awaited.
To summarise: the CMB data from WMAP and Planck is fully consistent with the standard six parameter Big Bang inflationary ΛCDM model. However there are anomalies in the tiny ripples of the CMB that are not predicted by the Standard Model and there is, as yet, no compelling explanation for the cause of these anomalies.
4.4 So, is the Universe inhomogeneous and anisotropic?
Of course it is. It is obvious that the Universe is both anisotropic and inhomogeneous on the scale of galaxies. A galaxy is, by its very nature, a gravitationally bound set of stars (and dark matter), which has a greater mass/energy density than the space between galaxies. In fact, it is clear, if one considers the distribution of galaxies from large galaxy surveys such as the Sloan Digital Sky Survey, that the Universe is inhomogeneous at least up to the scale of galaxy clusters and super clusters. Indeed it is inhomogeneous on the scale of the cosmic voids, sheets and filaments, which is known as the Cosmic Web, and which have structures at least 350 million light years across. According to the Standard Model, this structure arises from the same fluctuations that give rise to the anisotropies in the CMB.
So what do we mean by the Cosmological Principle’s tenet that the Universe is isotropic and homogeneous, if it is obviously not so up to scales of 350 million light years? The Cosmological Principle refers to the average distribution of matter and energy on the very largest scales that is used to make predictions about the long term behaviour of the Universe. The point is that the standard ΛCDM model already allows for inhomogeneities and anisotropies in the Universe, so it is only on scales beyond the transition to homogeneity at about 350 million light years[43] that the Cosmological Principle and the FLRW metric fully applies – otherwise it is an approximate description with perturbations due to the inhomogeneities of matter condensed into stars, galaxies and galaxy clusters.
5 The New Geocentrists’ claims
Bob Sungenis and Rick DeLano, with support from minor figures in the geocentricity movement such as Robert Bennett, Mark Wyatt and James Phillips, claim that the scientific observations that we have been discussing falsify the Copernican Principle, demolish modern cosmology and prove geocentrism.
I can do no better to convey what they say and how they say it than to let them speak for themselves:
- Everything we think we know about our Universe is wrong – Trailer for The Principle Movie
- What did COBE, WMAP and now Planck show us? Astounding as it may seem, the data reveals the Universe is non-Copernican, that is, it is not homogeneous as predicted by the Big Bang. There are warm and cool spots all over the Universe, which means the Universe is defined, with special locations and directions.
- They showed us that if we draw lines that connect all these warm and cold spots …those lines would point like an arrow from the edge of the Universe directly to the Earth
- The warm and cool spots of the CMB are systematically organized into distinct regions of the Universe that, when graphed on an X and Y axis, point directly to the Earth as the center of the distribution… It is very special and was placed in a very unique place in the Universe – in the very center.
- …the Big Bang – that is on the operating table taking its last breaths before it dies. And the Planck probe just put the knife into its heart
- So, even after they added in the fudge factors of Inflation, Dark Matter and Dark Energy for 96% of the Universe, the Planck probe comes back and says, “Sorry, the data from the Universe doesn’t fit your model. Go back to the drawing board and try again.”
- You know the old saying, “three strikes and you’re out.” Well, that is the case with Krauss and the rest of the scientific world. They have no place else to go.- Sungenis[44]
- …the CMB shows that the whole Universe is centered on the Earth. – Sungenis[45]
- It was so puzzling that NASA sent up the COBE probe in 1990 to take more measurements of the CMB. Lo and behold, COBE reported that the CMB was aligned with the Earth and the Sun. They couldn’t believe their eyes. So they sent up another probe in 2001 called the Wilkinson Microwave Anisotropy Probe (WMAP). You guessed it. WMAP came back with the same data – the Earth and Sun were aligned with the entire known Universe. – Sungenis[46] [47]
- The most shocking image I have ever seen, of Earth in the center of the Universe, is found on page 9 of the below new paper by Rubart and Schwarz – DeLano [48]
- Newest Evidence Shows Earth At the Center of the Observable Universe – DeLano[49]
- The Universe’s largest visible structure is aligned in completely inexplicable ways with supposedly insignificant Earth. This is now established, scientific, observational fact.– DeLano
- At the same time the alignment (aka: “axis of evil”) is an incredibly strong and compelling piece of evidence pointing to a geocentric universe! – Phillips[50]
- With COBE we saw that the CMB was aligned to the earth’s equinox and ecliptic, but science said bah, not possible. So we sent out the WMAP. The WMAP more clearly showed that the CMB is aligned to the earth’s equinox and ecliptic, but science said, bah, bah (and inside oh crud). So we sent out the Planck, using a different sensor and scanning methodology. Well Planck has once again verified that the supposed most primal signal in the Universe knows about is pointing directly at the earth.– Wyatt[51]
- Doesn’t anyone realize that the universal Cosmic Microwave Background has local axial and planar symmetries only when viewed from Earth? Doesn’t any scientist on this planet realize that it isn’t a planet? When will our stiff-necked scientists bow their heads and acknowledge the elephant in the living room, the emperor with no clothes? – Bennett[52]
That’s more than enough to give you a flavour for the lurid rhetoric and the reckless content of the geocentrists’ arguments. In many cases, they also reveal a fundamental misunderstanding of the science or the methods, which are too tedious to address individually. Contrast the tone of their writing with the obsessively detailed and careful discussion that can be found in any of the scientific papers referenced in this article.
5.1 Geocentrists’ misunderstanding
The geocentrists are clearly wedded to an a priori position, which is that the earth is the unmoving, non-rotating centre of the Universe. That is non-negotiable for them and they exploit any argument that they believe remotely supports their case. They play down all others. That’s not the way proper scientific discourse works. Rather than following the evidence where it leads, they are determined to wrestle the evidence into a shape that appears to support them. Reading the quotes above, one is struck by their categorical assertions and the extreme language in which they express them. Do the findings warrant their conclusions? Do they actually understand the scientific observations or are they relying on some cartoon version of them that they misinterpret in their favour?
Let’s look at the geocentrists’ position regarding their private story about cosmology and the CMB measurements (and really about Enlightenment science in general). For them, cosmology (indeed all of physics from Copernicus onwards) is a huge modernist conspiracy to displace the Earth in the minds of the public from what they see as its rightful place in the centre of the cosmos. They believe that “inconvenient” discoveries are swept under the carpet to maintain the conspiracy of silence that the science community has erected, and that in their movie, they are tearing down this veil for everyone’s benefit. As far as they are concerned, science in general and physics in particular is an atheistic plot to undermine Man’s special place in the universe. Well, if the disciplines of cosmology and physics are conspiring with atheists in this way, it must be the most incompetent and impotent conspiracy of all time. What sort of conspirators rush to publish “inconvenient” findings as soon as they are discovered and openly discuss their implications for the conspiracy? The geocentric crew would have no idea that the CMB has anisotropies and that some of those anisotropies are anomalous if it were not for the scientific publications of the very same people they regard as conspirators. For heaven’s sake, they wouldn’t even be aware of the existence of the CMB without them.
A further problem for geocentrists is that they understand neither the way physics as a discipline works nor the details of the physics they try to discuss. For example, Sungenis lambasts cosmologists for modifying the Big Bang model by introducing inflation, dark matter and dark energy 44 45 but fails to acknowledge that modifying the hypothesis to take account of new observations is how science works. People don’t propose overarching scientific theories out of the blue that are absolutely correct in every tiny detail like some vast infallible revelation. Our understanding of the Universe is gradually improved, step by step by a few clever ideas, a vast quantity of hard labour and false starts, dead ends, and breakthroughs. Physics and cosmology is a work in progress, and the fact that the Standard Model has evolved over time to accommodate new observations is a crucial strength – going where the evidence leads is what makes science the best way that we know to understand the natural world.
As for the geocentrists not understanding the science itself, two examples will suffice. Sungenis writes: “…how does Big Bang cosmology then explain the quadrupole/octupole axis, which is perpendicular to the dipole axis? It cannot be created by a movement of the sun-earth system through the CMB since, obviously, the sun-earth system cannot be going in one direction to create the dipole and, at the same time, going in an orthogonal direction to create the quadrupole and octupole. Something is definitely amiss here.” [53]
What’s amiss is Sungenis’s understanding of the CMB anisotropies – no knowledgeable person suggests that the quadrupole, octopole and higher multipoles arise primarily from motion of the solar system. As we have seen in Sec 2.4, they are the decomposition into spherical harmonics of the CMB anisotropies caused by Gaussian, nearly scale invariant fluctuations in the early Universe. By pointing out this misunderstanding I am not picking nits but pointing to a basic foundational lack of understanding on Sungenis’s part.
DeLano writes in [48], “The most shocking image I have ever seen, of Earth in the center of the Universe, is found on page 9 of the below new paper by Rubart and Schwarz”. That paper is reference [76] of this article. The only image to be found on page 9 of that paper does not merit hyperbole of that order and it obviously doesn’t show what DeLano thinks it shows. See Fig 9.
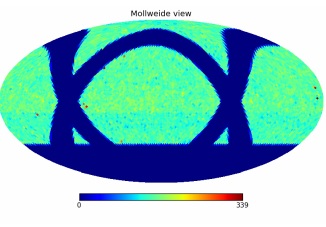
Fig 9: The most shocking image Rick DeLano has ever seen; from page 9 of ref [76]. The diagram shows number counts of radio galaxy sources from NVSS in equatorial co-ordinates. The dark blue solid lines are areas where there is no data or data has been masked out to prevent contamination from the Milky Way galaxy. Source: Rubart & Schwartz76
The geocentrists’ key misunderstanding of the science, which is the foundation for their most vociferous claims, is their misinterpretation of the directional alignment of the multipoles as data which yields positional information. The alignments, anomalous as they are, carry no information about the location of the Earth or any other object. We’ll explore this point in more detail below.
5.2 Are the geocentrists’ claims warranted?
In a sense this is the core of this article and what comes before serves as a preamble to prepare readers for this part of the discussion. The fact that there are unexplained anomalies in the CMB is undeniable and no-one is seeking to deny it. But the key question is whether these anomalies warrant the rhetoric and extreme claims of the geocentrists. There are four classes of argument used by them that we will consider in turn.
5.2.1: Geocentrist argument #1: “The CMB alignments point straight at the earth and therefore the earth is at the centre of the Universe.”
Although the approximate alignments of the dipole, quadrupole, octopole and ecliptic/equinox are not predicted and are currently unexplained, they do not “point like an arrow directly at the earth” and they are not evidence for geocentrism. As we have seen, the alignment is far from exact – the quadrupole vector is 23.1° from the equinox and 16° from the ecliptic plane, and the dipole is 28.5° from the quadrupole (Planck data, SMICA component separation, kinetic quadrupole corrected). Moreover, the geocentrists have never explained in detail how this approximate alignment of the low multipoles of the CMB with the ecliptic and the equinox is evidence for the earth being at the exact centre of the Universe.
It is clear that the geocentrists rely on a caricature of the science to make their claims. Sungenis misinterprets the approximate alignment as an arrow (or a sword in another case) pointing straight at the Earth. Wyatt claims that “the supposed most primal signal in the Universe knows about is pointing directly at the earth” (sic). DeLano writes “The Universe’s largest visible structure is aligned in completely inexplicable ways with supposedly insignificant Earth”.
In all of these quotes, the geocentrists interpret the vectors that define the orientation of the multipoles to be carrying positional information. In other words, they imagine that the multipole vectors are lines which pass through the Earth, and coincide at the Earth in a unique manner. They imagine that there is no other planet or star or other body in the Universe where the vectors coincide, and that therefore they uniquely point to the Earth’s location. However, their understanding of the vectors is simply wrong. The vectors define directions but carry no information about location. They are not unique lines on which the Earth is located. They don’t point to any location.
To illustrate this, let’s think about a crystal, which is a structure that has a number of well-defined planes. For example, rock salt has cubic crystals[54]. The planes of a crystal structure are analogous to the CMB multipole planes and the directions orthogonal to those planes are analogous to the multipole vectors. Imagine being somewhere in a vast salt crystal. You would be able to measure the orientation of the crystal planes (and they would be the same wherever in the crystal you were), and define those orientations by vectors orthogonal to the planes but the directions in which the vectors point would give no positional information. They cannot be used to define a location in the crystal or to navigate to a specific location. Similarly, the CMB multipole vectors give directional information but no positional information. If you were an astronomical distance away from the Earth, you would not be able to use the CMB multipole vectors to navigate to it.
What’s more, most of the planets in our solar system have orbital planes that align with the quadrupole and octopole to much the same degree that the Earth does. In fact, because the alignment of the multipoles with the ecliptic is only approximate, the evidence strongly indicates that there is a vast number of planets in our galaxy (as well as an unimaginably large number in other galaxies) that have orbits that align more precisely with the quadrupole and octopole than the Earth does. The anomalous CMB alignments simply do not distinguish the Earth in the way the geocentrists imagine that they do, and they certainly don’t point to it as being in any way central.
Let’s think about this in another way. Let’s consider the nature of the CMB anisotropies. As we have seen, they arise from density fluctuations in the early universe that are seeds for the current structure of galaxies and galaxy clusters. If the alignment of the low multipoles is “pointing straight at the earth”, then we would expect the quadrupole and octopole to be aligned at the earth and for that alignment to reduce rapidly as we move away from the Earth. If they don’t behave like this then they cannot distinguish the Earth’s location. But the co-moving size of the structure that gave rise to the octopole is 29 billion light years across, and the quadrupole is bigger than that, so the alignment is the same a very long distance away from the Earth; in fact a very long distance away from our galaxy group. There would be no way of distinguishing the location of the Earth from elsewhere in the Universe by measuring the direction of the CMB vectors. The idea that the alignment is a signal pointing at the Earth is based on a misunderstanding of the nature of the primordial CMB anisotropies. That misunderstanding is profound, but it is not one that the geocentrists are likely to correct quickly because they have invested a great deal of their rhetoric in it.
Neither Sungenis nor any of his colleagues can explain in detail just how the observations, including the alignments, support geocentrism. Let’s assume that the alignment of the low multipoles with the ecliptic is caused by some as yet undiscovered physical effect, either local or cosmological as the geocentrists prefer – let them give us an example of what they think that effect might be, and how it causes the alignment of the low multipoles consistent with everything else that we know about the Universe, explaining in detail how the effect, to the approximate degree that is measured, leads to the conclusion of geocentrism. Without hand-waving or table thumping can they tell us precisely how this alignment is evidence that the earth is unmoving in the centre of the Universe? Of course they can’t. In order to do that, they must wind back to first principles by defining the origin and nature of the CMB in a way that is consistent with detailed measurements such as the anisotropy power spectrum of Figure 7. If you look at any scientific paper referenced in this article (references are generally to the arXiv pre-print server, which is open to all – unlike some other areas of science, this “conspiracy of cosmology” puts all its papers in free public view and not behind a pay-wall), you will find that astrophysicists and cosmologists engage in discussion that is detailed and quantified almost to the point of obsession. The challenge to the geocentrists is to stop leaping to conclusions and to explain in detail what the source of the CMB is in their cosmological model[55], what physical effects lead to the approximate alignments we observe, and how those effects and those alignments provide positional information and evidence for geocentrism.
It is worth noting that geocentrists are exercised by their tenet that the centre of the universe is precisely at the Earth. Both WMAP and Planck, which made the CMB measurements, were located at the Sun-Earth Lagrange point, L2, almost a million miles from the Earth. In their model does that mean that the centre of the Universe is at the Sun-Earth L2, a million miles from the Earth?
5.2.2 Geocentrist argument #2: “The CMB alignments point straight at the earth and therefore the Earth is in a privileged or unique place.”
This is a similar (but less extreme) claim to #1. Similar arguments apply – to be credible, the geocentrists should identify the source and nature of the CMB, identify the effect that is causing the alignment and explain how the operation of that process and the actual measured data leads us to conclude that the Earth is in a privileged or unique place.
As we have seen, the alignment of low multipoles changes extremely slowly with distance away from our galaxy because of the size of the structures that give rise to them (remember that the octopole has only eight lobes and the quadrupole just four lobes on the entire sky) – the low multipoles represent vast structures in the present universe. So the CMB alignments would be the same not just at the Earth, but anywhere within a few billion light years of the Earth. They give directional but not positional information.
5.2.3 Geocentrist argument #3: “There are anomalies in the CMB anisotropy, and therefore the Cosmological Principle is false, therefore the Copernican Principle is false (therefore the Earth is at a privileged place).”
Let us remind ourselves that the CMB is uniform to ten parts in a million, and that the CMB anisotropies arising from the Gaussian, nearly scale invariant fluctuations that eventually led, through the evolution of structure in the Universe, to the galaxy and galaxy group structure that we currently observe, were predicted long before they were actually measured[56] [57]. The anomalies in the CMB anisotropy, and other measurements that indicate some large scale anisotropy in the Universe (we’ll look at some of these more closely in Section 6 below), such as they are, can be regarded as perturbations on a broadly homogeneous and isotropic Universe. So far no anisotropies or inhomogeneities have been uncovered that are of a magnitude that preclude them arising from physical processes in the early Universe. In addition to the uniformity of the CMB, large scale galaxy counts indicate that the Universe is largely homogeneous above the 350 million light year scale43. Measurement of the average bulk flow of galaxies against the CMB rest frame, using data from the Planck satellite, indicates that there is no average flow, which is strong evidence for homogeneity on the scale of billions of light years[58].
The CMB anisotropies are predicted in detail by the inflation ΛCDM model and that model predicts structure on very large scales – in fact the quadrupole, octopole and some cosmic portion of the dipole are the signal for this large scale structure on the surface of last scattering. The structure is present everywhere in the Universe including within the observable part of the Universe in which we live. Therefore it is possible that, on the largest scale, we live in an over or under-density that arises from the same mechanism as the quadrupole, octopole and other large scale multipoles. The consequence is an inevitable uncertainty in how the large scale CMB multipoles appear to us – the very fluctuations that are being measured will result in the Universe appearing slightly different to observers in another place, on about the same scale, but there is no way of determining purely from the CMB anisotropy spectrum where within the structure we are. This uncertainty is called cosmic variance and is greatest at low multipoles. It is illustrated in Fig 6 and Fig 7 by the shaded portion towards the left hand side of the graphs. Cosmic variance is a source of cosmic anisotropy within a basic ΛCDM model. We’ll return to this subject in Section 6 below.
But suppose these anomalies are caused not just by cosmic variance but are pointers to some large scale and cosmic anisotropy or inhomogeneity in the Universe of a magnitude that fundamentally challenges the Cosmological Principle. Suppose the Universe is substantially different at different places and in different directions. The consequence of this for cosmology would depend in what way it departs from perfect homogeneity and isotropy and by how much. What does any violation of the Cosmological Principle mean for the Copernican Principle? Suppose it is so much violated that we have to abandon the FLRW metric for some other mathematical description at the cosmological level. Well, even if the Universe is substantially inhomogeneous and anisotropic on the largest scales, that does not lead to the conclusion that the Earth is in any special or privileged place and so we could not conclude from that the Copernican Principle is violated.
The observation stands that the Earth is a planet orbiting one of billions of ordinary stars located in an unremarkable place in the spiral arm of an ordinary galaxy, which is one of billions of galaxies. That observation has not been modified by any recent observations.
In order to demonstrate that a violation of the Cosmological Principle results in the Earth being a special or privileged place, one needs at least a clear definition of what is meant by being in a special or privileged place. Well, at least we know what the New Geocentrists mean by it – they mean that the Earth is physically static and at the precise centre of the Universe. Violations of the Cosmological Principle in themselves are not evidence for this.
By the way, what the geocentrists need to support their ideas is a Universe that is inhomogeneous but which appears isotropic from the point of view of the Earth, because they think the Earth is static at the centre. If I were a geocentrist, I’d be rather worried by these CMB anomalies. In general the anomalies are challenges to large scale isotropy. If we were literally positioned at the centre of a finite, bounded, spherically symmetric, flat Universe (the sort of Universe the geocentrists believe in), then we would observe isotropy, even if the Universe is inhomogeneous (which it would be bound to be if it is finite and bounded). Anisotropies of the kind that might cause the observed anomalies would exist away from the centre and not at it, so these anomalies are evidence against their position rather than in support of it.
5.2.4 Geocentrist argument #4: “There are anomalies in the CMB anisotropy, and therefore the Standard Model of cosmology must be abandoned.”
As we have seen, the CMB data is in excellent agreement with the Big Bang inflation ΛCDM model. The detailed prediction for the CMB anisotropy spectrum has been met very closely by the observations and rather than demolishing the model, it has provided excellent evidence in support of it. The anomalies appear to be secondary unexplained features on the overall structure. The overall structure, in most respects, matches very well. Therefore the approach that most cosmologists are taking is to continue working with the Standard Model while seeking explanations for the anomalies in the CMB data and in other observations. At the moment there is no reason to think that it will be impossible to explain the anomalies by refining the vanilla Standard Model to incorporate some currently unrecognised process, which might or might not involve new physics.
Of course, in the future it is possible that these anomalies, along with further observations, will falsify some crucial aspect of the Standard Model, and then cosmologists will have to seek something better. At the moment however, the Standard Model, including inflation, dark matter and dark energy predicts what we see very well, and has received improved support from Planck and WMAP data, and so it remains an excellent cosmological model, and the best currently available.
The Universe does not have to conform to our preconceptions. There is no guarantee that the physics of the Universe is simple, and no reason to think that we won’t continue to discover more about the details the closer we look. Geocentrists project their own way of doing “science” on to professional scientists. They are wedded to a particular a priori conclusion and so they think the professionals are the same. They fear and ignore evidence contrary to their conclusion, so they believe that the professional must do the same. In fact, cosmologists and astrophysicists welcome novel signals (and the anomalies can be regarded like that) that lead to better understanding of known processes or the discovery of new processes. If you read the primary literature, you’ll find that that is the tone of the discussion about the detection of the anomalies – people are intrigued and excited by what they might mean rather than fearful that they might destroy some cherished theory.
5.3 The geocentrists lack a model
Sungenis, DeLano and their friends are very good at sitting on the side-lines and throwing bricks at the scientific community but they haven’t felt the need to create a model of their own. To the extent that they do research, it is by textual exegesis or literary analysis of scientific papers, lacking as they are in mathematical skills, the real language of physics. I covered this briefly in Sec 5.2.1 above, but we’ll look at it in more detail here. If you read the books and papers promoted on their websites and blogs (Galileo Was Wrong, Magisterial Fundies, geocentrism.com and so on), you will find that they are quite antagonistic to science in general, particularly physics after Copernicus and biology after 1859. Concentrating on cosmology and astrophysics, here are some of the things that they are opposed to: the Big Bang, an old Universe, a Universe bigger than a few thousand light years across, inflation, Special Relativity, General Relativity, quantum physics, the expansion of the Universe, redshift correlations with distance, Newtonian gravity on extra-galactic scales, the abandonment of the luminiferous Aether, an infinite Universe, an unbounded Universe, curved spacetime, non-Euclidean geometry of space, dark matter, dark energy and so on. (Of course their opposition is based entirely on blind prejudice since they don’t possess the mathematical skill or grounding in physics to understand or properly assess what they reject).
They spend much of their energy impotently opposing one or other of these concepts (quite often using one, which they deny, as an argument against another, which they are trying to deny – consistency is not their strong suit) but very little energy, indeed none at all, in developing a model that is cosmologically and astronomically valid and self-consistent, and which supports their assertions. Such a model should explain all of the huge quantity of observations of the cosmos, from the linear relationship between distance and luminosity of standard candles, such as standard Cepheid variables, to the detailed measurements of the CMB that we have been discussing here. Their theory should explain the flat rotation curves of galaxies, the non-proportionality in redshift and luminosity of Type 1a supernovae, the relative abundance of the elements, the baryon density, and the structure of the Universe as far as stars, galaxies and galaxy clusters are concerned. They would have to explain the development of the early Universe and have a good solution for the Horizon, Flatness and Magnetic Monopole problems. Presumably they would need a new theory of gravity since they reject Special and General Relativity (and their novel theory of gravity would also have to be consistent with observations and all the tests that SR and GR have passed[59]). They would need to do all this with a model that is self-consistent (so it must have no major internal inconsistencies and contradictions), quantified (so it would have to be mathematically based) and provide at least the same, and preferably better, quantifiable predictions as the model it replaces (so for example it would have to predict the first peak in the CMB anisotropy power spectrum at l=220 plus the location of the other peaks).
That’s a tall order for a handful of people who mostly have neither physics education nor mathematical skill. After all, the Standard Model has been developed by tens of thousands of professionals over more than a hundred years. You might accuse me of being unfair and you’d be right. We shouldn’t expect them to be able to do this. But on the other hand, if they can’t even set out the bare-bones framework of such a model that doesn’t fall at the first empirical hurdle, and which has at least the potential to meet the criteria above, how do they know that the standard model that they are opposed to is as wrong as they say it is?
So, let’s narrow down our challenge somewhat. Let’s ask a specific question about the CMB anomalies that the geocentrists should be able to answer if they are in a position to support their claims with evidence and logic. How precisely do the anomalies of the CMB support the geocentric argument? It’s not good enough to point to current unexplained observations, and from there to jump with a hand-wave to whatever conclusion they want. “Scientists don’t currently know why the quadrupole and the octopole align approximately with each other and on the dipole and ecliptic therefore the Earth is at the centre of the Universe” is not a compelling argument, but it is basically the argument that they are making. ““Scientists don’t currently know why the quadrupole and the octopole align approximately with each other and on the dipole and ecliptic therefore the Earth is at a privileged or special place” isn’t any better.
The question to the geocentrists is: if the Universe is rotating daily about the Earth fixed at its centre, on an axis running through the terrestrial poles (i.e. on the equatorial plane) as they believe, why would we expect the large scale multipoles of the CMB anisotropy to be aligned to each other and the ecliptic? To answer the question they need to come up with a plausible hypothesis for the source of the CMB, which is consistent with its temperature and its black body spectrum, and which makes a stab at predicting the richness of the observed statistics of its anisotropy. That hypothesis should include a quantified explanation of how the daily rotation of the Universe about the fixed earth at its centre on the plane of the Earth’s equator leads to the approximate alignment that we observe between the low multipoles, the dipole and the ecliptic. At the very least it should explain quantitatively how the observed alignments are evidence for a central Earth.
Is that also too difficult? Well, perhaps they could demonstrate that the anomalies are such that the FLRW solution to the Einstein field equations is an unacceptable approximation[60] and that cosmology should be based on a different metric? In doing so they would need to start with their explanation of the nature of the CMB and its anisotropies as before, derive the quantified cosmological consequences of the anomalies and show that those consequences result in a metric that is significantly different from that given by the Friedmann equations. This is what any scientist who claims that the Universe model should be based on a different metric would be required to do. Why not Sungenis, DeLano and friends?
In conclusion, the geocentrists lack a self-consistent, coherent cosmological model. Their arguments are all based on ad-hoc considerations and they are unable to point out, beyond vague hand-waving, how the anomalies that they make such a song and dance about are actually evidence for their case. Their claims are unwarranted.
6 Looking beyond the CMB
Although we have focused mainly on the CMB anomalies in this review, there are some other considerations that are relevant to the question of whether the Universe is homogeneous and isotropic that we will consider in this section.
6.1 Alternatives to dark energy – cosmic voids
You will remember from Section 3.5 that dark energy was proposed to explain the apparent acceleration of the expansion of the Universe and to make the total mass/energy density in the universe close to the critical mass. Almost at the same time as the hypothesis of dark energy was proposed, a number of other proposals were made to explain the supernova 1a data (the observation that the luminosity or brightness of type 1a supernovae dims more than expected with distance). The most interesting of these proposals and the one that has lasted longest is the idea that we live near the centre of a cosmic void[61] [62].
The idea is that what can be interpreted as accelerated expansion in a perfectly homogeneous universe, can also be interpreted as being caused by the observer being near to the centre of a large under-dense region of the universe, which would imply that the universe is inhomogeneous on at least that scale. This proposal neatly avoids the need for dark energy and is therefore being actively explored.
Observations, such as the uniformity of the CMB, indicate that we would need to be within 15Mpc (or about 50 million light years) of the centre[63] of such a void (or further away from the centre but moving towards it). It has been suggested that being off-centre in such a void can explain some of the anomalies of the CMB anisotropy (alignment of the low multipoles and asymmetry between hemispheres)[64]. The size of the putative void has been constrained by observations of a particular physical phenomenon called the kinetic Sunyaev-Zel’dovich effect to be less than 4.5 billion light years, which is smaller than the observable universe[65]. In order to match other observations, such as the position of the first peak in the CMB anisotropy power spectrum, the Universe outside the under-dense region would have to be homogeneous on the scale of the cosmological horizon[66] and above. A large number of other papers have been published on this subject including reviews[67].
Astrophysicists and theorists have been developing mathematically based models of the universe that represent the void in order to compare their predictions with observations. The most common of these models describe a region that is spherically symmetric but inhomogeneous, and expanding or collapsing under the influence of GR gravity. To model the void, they use a spherically symmetrical and inhomogeneous solution to the Einstein field equations called the Lemaître-Tolman-Bondi (LTB) solution that was first proposed by Richard Tolman in 1934[68] and further developed by Hermann Bondi in 1947[69].
The models of the Universe with a void comprise an inhomogeneous spherical region or void in an otherwise homogeneous universe. The homogeneous background is represented by the FLRW metric that we discussed earlier, confined to matter only and flat space (this constrained version of the Friedmann solution is also known as an Einstein-deSitter space). The transition between the LTB and the Einstein-deSitter space has been modelled in a number of ways[70]. There also exists a hypothesis in which a series of spherical shells of increasing radius, each shell having different matter density, blend into the homogeneous flat universe beyond the void[71].
So far, observations do not definitively favour the Standard Model with accelerating expansion over an inhomogeneous cosmic void. However, recent measurements do favour the Standard Model and disfavour cosmic voids, and the most likely varieties of cosmic void have been excluded. A recent direct measurement of cosmic homogeneity using the WiggleZ spectroscopic survey of 200,000 galaxies[72] favours the Standard Model. More recently, the BOSS experiment, which uses the preferred spacing of galactic structures based on the huge Sloan Digital Sky Survey III, more strongly favours the Standard model[73]; in other words BOSS is compatible with a homogeneous universe undergoing accelerating expansion. We’ll discuss the BOSS experiment in somewhat more detail below. Even more recently, a study by the Planck team of the motion of galaxy clusters in the search for an average bulk flow against the CMB rest frame concluded that no such flow existed. Moreover the data rules out adiabatic voids that have sufficient depth and size to correctly predict the Sn1a data58. Measurements of the Hubble constant yield a value that is generally higher than is predicted by the void models[74] and therefore disfavour the void hypothesis. We can therefore say that although void models proposed to explain the Sn 1a data are not currently ruled out, they are highly disfavoured by observations.
The geocentrists’ reaction to these suggestions is typical sensationalism. They claim that if a void turns out to be the cause of the dimming of distant type 1a supernovae then this will disprove the Cosmological Principle and with it the Copernican principle and the Big Bang. Furthermore they claim that since many of the void models constrain our current location to be near the centre of the void then that is evidence or proof that we are in a special place or even that we are at the centre of the Universe.
This rhetoric is highly exaggerated. Even if the explanation that we live near the centre of a cosmic void becomes accepted in the future, that will not ipso facto invalidate the Big Bang. In the void models, the voids are imbedded in a larger surrounding homogeneous space and that, and the fact of ongoing expansion of the Universe, would continue to support Big Bang. Physicists would, of course, wish to explain how the void arises within a Big Bang scenario. However, the Big Bang is not incompatible with the existence of a cosmic void of the kind proposed. In fact a number of proposals exist[75] for how such a void can arise as a result of primordial anisotropies within a Big Bang model.
The geocentrists’ claims that the void proposal is evidence for or proof that the Earth is in a special place or even that the Earth is at the centre of the Universe are even more greatly exaggerated. The void hypotheses only require the Earth to be near the centre of the void (and ‘near’ in this context means less than 50 million light-years – or 500 Milky Way diameters, so not really that near; the geocentrists claim that the Earth is the exact centre of the universe, and the Sun, 8 light-minutes away, is not at its centre. I’m sure readers can see that 50 million light-years is a lot more than 8 light-minutes) and being near the centre of the void does not mean the same as being near the centre of the Universe. The proposition that the dimming of the SN1a supernovae arises from our location within 50 million light years of the centre of a cosmic void or under-density, even if that idea were to be more certain than an unconfirmed hypothesis, is not evidence for geocentrism.
6.2 The puzzle of the radio galaxy dipole amplitude
Radio galaxies are those that emit and are detected at radio wavelengths. Several very extensive catalogues of radio galaxies containing data on hundreds of thousands of galaxies have been gathered. Galaxies do move with respect to one another – in fact the velocity of nearby galaxies with respect to our own can be readily measured, and the velocity of the Milky Way galaxy with respect to the CMB rest frame is one component that creates the CMB temperature dipole. However, in a homogeneous universe, we would expect the mean velocity of a large number of galaxies in all directions of the sky to be zero with respect to the rest frame of the CMB, so the mean velocity of the solar system with respect to the radio galaxies should be the same as it is with respect to the rest frame of the CMB as derived from the CMB dipole. Several analyses have been carried out to measure the mean radio galaxy velocity[76].
The method of measuring the mean velocity of the radio sources is not what you might think. The Doppler shift of each galaxy is not measured directly, but the galaxies above a certain arbitrary threshold of flux are counted. In the direction of motion of the solar system we should find more galaxies above the threshold (since the energy of the photons is increased by Doppler shift) than in the opposite direction (where the energy of photons is reduced by Doppler shift). And indeed all the analyses carried out, bar one, yield a result where the direction of the motion with respect to the mean of the radio galaxies is in the same direction (within errors) as the CMB dipole. That is exactly as expected.
What is unexpected is that the detected velocity ranges from two to five times the velocity measured from the CMB[77]. As yet there is no agreed explanation for this. Does it reflect anisotropy in the universe on the scale of the current surveys? It is possible that it arises as an artefact of assumptions made in the analysis about the spectral characteristics of the radio sources, the statistical distribution of radio intensities and physical distribution of radio galaxies which is known to be inhomogeneous and anisotropic on medium scales. Another possibility is that it arises from an average flow of galaxies with respect to the rest frame of the CMB within the scale of the survey.
It is also possible that the discrepancy in the velocity of the solar system inferred from the CMB and the radio galaxy dipoles can be explained in the context of a local under- or over-density such as we have discussed before[78]; such a possibility is discussed in a paper that has recently been made available[79]. In this case, some of the dipole can be explained by the existence of a void rather than being a result of the motion of the observer – in other words a void would result in a radio galaxy dipole that depends at least partly on cosmic structure rather than the motion of the observer. Such a result could arise for cases where the observer is either inside or outside the void.
It is surprising that the New Geocentrists think that this is good news for their case. Whether or not these data ultimately support the conclusion that the Universe contains features that are unexpectedly inhomogeneous, the existence of a large radio galaxy dipole (and anisotropies in the measurements of polarisation direction of quasars[80], indeed any anisotropy on a large scale) is evidence against the idea that the Earth is at rest in a central or privileged place, in which scenario one would expect the Universe to look identical in all directions.
6.3 Periodicities in galaxy redshifts
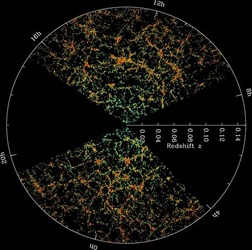
Fig 10: Slices through the SDSS 3-dimensional map of the distribution of galaxies. Earth is at the center, and each point represents a galaxy, typically containing about 100 billion stars. Galaxies are colored according to the ages of their stars, with the redder, more strongly clustered points showing galaxies that are made of older stars. The outer circle is at a distance of two billion light years. Both slices contain all galaxies within -1.25 and 1.25 degrees declination. Credit: M. Blanton and the Sloan Digital Sky Survey. http://www.sdss.org/
The idea that galaxies are arranged in shells at preferred distances around the Earth and that therefore the Earth is at the centre of a vast large-scale structure has been proposed for some time (this idea is known as redshift quantisation or periodicity). Standard cosmology predicts that the galaxy clusters would condense into large scale strings and nets, called the Cosmic Web, and indeed that is what is observed (see Fig 10). It seems to be the case that early reports of redshift quantisation based on relatively small catalogues of galaxies, mistook the local galactic network as evidence in support of periodicities. Once extensive galaxy surveys became available, the idea that the distribution of galaxies and galaxy clusters are quantised and centred on the Earth has been shown to be false[81] or at least not well established[82].
Nevertheless we need to ask whether there is any sort of preferred scale or structure in the galaxies. Referring back to Sec 2.4 let’s remind ourselves of the acoustic waves in the early universe known as Baryon Acoustic Oscillations. You will remember that these BAOs intensified the primordial anisotropies in the CMB at certain scales and suppressed them at other scales, and this is the explanation for the existence and position of the first peak in the CMB anisotropy power spectrum (Figs 6 and 7). The fluctuations in the density of matter at the time of decoupling, which were the cause of the CMB temperature anisotropies, were also seeds for the gravitational condensation of matter into galaxy clusters and superclusters. We therefore expect to see structures in the current universe that have preferred dimensions corresponding to the peaks of the CMB anisotropy. The preferred dimension for the first peak in the CMB when converted to the current universe by applying the expansion factor of the universe since decoupling is about 150Mpc or about 500 million light years. The Sloan Digital Sky Survey III experiment, Baryon Oscillation Spectroscopic Survey (BOSS)[83], was designed to detect and measure this effect. BOSS finds that the structures in the universe have preferred scales in good agreement with the BAO model, in other words, galaxies are clustered at preferred scales (but at randomly located centres – BAO structures are not the same as redshift periodicity) predicted by the physics of the acoustic oscillations[84]. By measuring the dimensions of the preferred scales for structures a long distance away, and therefore a long time ago, BOSS is able to measure the rate of expansion of space in the past and therefore determine whether the universe is undergoing accelerated expansion. BOSS is therefore an independent check of the conclusion based on the Type 1a supernova distance-luminosity data that indicated accelerating expansion. The BOSS data supports accelerating expansion and is entirely consistent with the hypothesis of dark energy.
In 2007, John Hartnett and Koichi Hirano carried out a Fourier analysis of the redshifts of galaxies in the 2dF GRS and SDSS galaxy survey catalogues, and claim[85] that there is a detectable periodicity in the number of galaxies at particular redshifts. This could indicate that galaxies are preferentially located on concentric shells with dimensions corresponding to those redshifts assuming an approximately constant Hubble expansion. Hartnett and Hirano suggest that the redshift periodicity (which appears in redshift space rather than distance or wavenumber space[86]) might be caused by oscillations in the expansion rate of the past universe, a hypothesis which Hirano is pursuing in the literature[87]. Bell and Comeau[88] have reported similar apparent oscillations in the expansion rate based on Type 1a supernova data.
The interpretation of the data by Hartnett and Hirano85 has been challenged on-line by Tom Bridgman[89]. He claims that the existence of enhanced spatial frequencies in their one-dimensional analysis of a three-dimensional data set does not mean that the data is periodic; and that the proper way to carry out a spatial frequency analysis on a 3-D data set is to perform a full 3-D transform on the entire data set. Interestingly, Hartnett has reported[90] that apparent periodicity in quasars from the Sloan Digital Sky Survey is not real but is a selection effect – in other words it is caused by an artefact of the measurement or analysis.
Setting aside Bridgman’s objections for the sake of argument and accepting that the data does support the periodicity hypothesis, and assuming it is caused by oscillations in the expansion rate of the universe in the past, as proposed by Hartnett and Hirano, let’s consider what implications that has for geocentricity. In that case, observers will see preferential clustering of galaxies as a function of redshift, in concentric shells exactly centred on themselves from wherever in the universe they make the observation. Just like the recession of galaxies in a uniformly expanding universe always appears to be exactly centred on the observer, so universal oscillations in the expansion rate would leave a signature in the redshift data precisely centred on the observer, wherever he is. At first sight it might seem strange that you can see the same effect of shells of increased density centred on yourself wherever you are, but we can understand this if we consider that we are looking back in time as well as looking across space when we look at galaxies a long way away. If the universe was expanding less slowly, say, 100 million years ago, then we would see an increased density of galaxies at the redshift corresponding to a look-back time of 100 million years (in other words we’d be looking at the density as it was 100 million years ago), which lies on a shell centred on us because it has taken 100 million years for the light from that time to reach us from all directions in space; and everyone else in the Universe will see the same thing. So, even if this controversial periodicity analysis is confirmed, it is not evidence that the Earth is at the centre or at a special place.
In the original paper, Hartnett and Hirano refer only to the oscillating expansion rate hypothesis. In a second sole-author paper[91], Hartnett explores the possibility that the periodicity in the data is caused not by an oscillating expansion rate but by anisotropic co-existing expanding shells of increased galaxy count. If that were the case the data would look different depending on where in the universe the observer is. Hartnett has calculated that the data supports this scenario, if the centre of the shells is 137 million light years away from us. Hartnett very fairly and clearly presents these alternatives on his blog[92]. Neither alternative supports strict geocentrism. According to the first hypothesis the same data would be obtained from any vantage point in the Universe and so provides no support for geocentrism; and according to the second, the centre of the shell structure is displaced from the Earth by 137 million light years.
7 Conclusion
I have reviewed the discovery, science and characteristics of the CMB. I have explored the Standard Model of the Universe and explained the rationale for the inclusion of its most important features. I have described the anomalies that have been discovered in the CMB and discussed their implications for cosmology. I have assessed the geocentrists’ claims against this information. Finally I have considered a few other observations that are anomalous or that call the Cosmological Principle into question.
I have shown that the geocentrists’ claims are not warranted by the science. Having started with a preconceived and unshakeable conclusion, geocentrists are engaged in a very unscientific process, mining for evidence that they believe supports their case or that they think they can serve up to an uninformed audience to persuade them that modern cosmology is in crisis and that geocentrism is set to replace it. Quite apart from the geocentrists’ histrionic tone, their conclusions are generally unwarranted, chiefly because they do not follow from the science. The geocentrists have no cosmological model of their own and they are unable to explain in detail how the observations support their case. On many occasions, they misunderstand or misrepresent the science.
The anomalies in the CMB, and the other observations that we have reviewed do not support strict geocentrism, and they disprove neither the Copernican Principle nor the Standard Model of Cosmology. We have seen that observations in the last decade have reinforced rather than undermined the Standard Model. Of course, that is not to say that the Cosmological Principle of homogeneity and isotropy on the largest scales is sacrosanct. Astrophysicists and cosmologists publish and discuss many challenges to that concept, but even in the extreme case, geocentrism is not the natural successor to the Standard Model, should a substantially inhomogeneous universe model become accepted.
The basic fallacy of the geocentrists is to believe and to argue that evidence against the Standard Model and against the Cosmological Principle, such as it is, is evidence in favour of a geocentric cosmology. It’s not.
End Notes:
[1] See for example http://galileowaswrong.com/
[2] http://www.theprinciplemovie.com/ and https://www.facebook.com/theprinciplemovie
[3] Here Comes the Sun, https://www.geocentrismdebunked.org/wp-content/uploads/2014/04/Here-Comes-the-Sun-Alec-MacAndrew.pdf
[4] The other major cosmological theory was the Steady State model supported by Fred Hoyle, Hermann Bondi, Thomas Gold and others. It did not predict and cannot explain the black body spectrum of the CMB, and so it has fallen away.
[5] The theory underpinning the behaviour of electrons and their interaction with light, Quantum Electrodynamics or QED, is the most precisely predictive theory in physics
[6] Zel’dovich, YB, A hypothesis, unifying the structure and the entropy of the Universe, Monthly Notices of the Royal Astronomical Society, Vol. 160, p. 1P (1972)
[7] There are two effects in play here: the photons in more dense regions are intrinsically hotter, but GR predicts that they are cooled as they climb out of the deeper gravity potential well of the higher density. The latter effect wins: it is 50% larger than the intrinsic effect as we perceive it. The result is that the cumulative temperature fluctuation is 1/3 that predicted by the gravitational effect alone
[8] For those of a technical and mathematical bent, unlike the discrete Fourier transform, which decomposes the signal into a series of sinusoids, the spherical harmonics of the CMB are a series of Legendre polynomials.
[9] For a mathematical treatment of this theory go to Ned Wright’s cosmology primer: http://ned.ipac.caltech.edu/level5/Sept02/Reid/Reid5_2.html
[10] The COBE scientific papers are here: http://aether.lbl.gov/www/projects/cobe/papers.html
[11] The huge set of technical WMAP papers can be found here: http://lambda.gsfc.nasa.gov/product/map/current/map_bibliography.cfm
[12] The ESA Planck mission reports here: http://www.sciops.esa.int/index.php?project=PLANCK&page=Planck_Published_Papers
[13] http://cmb.phys.cwru.edu/boomerang/
[14] http://cosmology.berkeley.edu/group/cmb/
[15] Planck Collaboration, Planck 2013 results. I. Overview of products and scientific results arXiv:1303.5062v1
[16] Big Bang Nucleosynthesis is the theory that predicts the relative abundance of the nuclei of hydrogen, helium and lithium, the light elements, in the early and current Universe
[17] There is also a third problem of quantum field theory solved by inflation – the prediction of magnetic monopoles, which have, so far, not been observed.
[18] Guth A H, “The Inflationary Universe: A Possible Solution to the Horizon and Flatness Problems”. Physical Review D23: 347 (1981)
[19] Adiabatic perturbations are those where the matter and energy fluctuations are in phase – in other words, the fluctuations would be in thermal equilibrium.
[20] Zwicky, F, Die Rotverschiebung von extragalaktischen Nebeln, Helvetica Physica Acta, Vol. 6, p. 110-127 (1933)
[21] See for example: Rubin et al, Rotation Velocities of 16 Sa Galaxies and a Comparison of Sa, Sb, and Sc Rotation Properties, Astrophys J 289, 81, (1980)
[22] The Baryon Acoustic Oscillation theory explains quantitatively why the distribution of dark matter in galaxies is different from the distribution of ordinary matter such as stars.
[23] Type 1a supernovae are white dwarf stars that accrete matter up to a certain critical mass known as the Chandrasekhar limit. At this point the star collapses and carbon fusion of most of the mass of the star takes place within a few seconds, blowing the star apart and releasing an enormous quantity of energy.
[24] Riess A et al. (Supernova Search Team) (1998). “Observational evidence from supernovae for an accelerating universe and a cosmological constant“. Astronomical J. 116 (3): 1009–38. arXiv:astro-ph/9805201
[25] Perlmutter S et al. (The Supernova Cosmology Project) (1999). “Measurements of Omega and Lambda from 42 high redshift supernovae“. Astrophysical Journal 517 (2): 565–86. arXiv:astro-ph/9812133
[26] The 2dF Galaxy Redshift Survey
[27] Sherwin et al, Evidence for dark energy from the cosmic microwave background alone using the Atacama Cosmology Telescope lensing measurements, arXiv:1105.0419v3
[28] The measurement of the CMB is not all down to WMAP and Planck: see for example Sievers et al, The Atacama Cosmology Telescope: Cosmological parameters from three seasons of data, arXiv 1301.0824v3
[29] Planck Collaboration, Planck 2013 Results. XXIII. Isotropy and statistics of the CMB, arXiv:1303.5083v3
[30] Ibid, Sec 5.2
[31] Planck Collaboration, Planck 2013. Results. XXVII. Doppler Boosting of the CMB: Eppur si muove, arXiv:1303.5087 where additional effects in the CMB (increased power in the anisotropies in the direction of travel and CMB aberration) are strong evidence that the dipole is caused by motion of the solar system relative to the CMB rest frame.
[32] Planck Collaboration, Planck 2013 Results. XXIII. Isotropy and statistics of the CMB, arXiv:1303.5083v3, Sec 5.3
[33] Ibid Sec 5.5
[34] Ibid Sec 5.8
[35] Ibid Sec 5.9
[36] See for example: Vielva, A Comprehensive Review of the Cold Spot, arXiv:1008.3051
[37] Bennett et al, Nine-Year Wilkinson Microwave Anisotropy Probe (WMAP) Observations: Final Maps and Results, arXiv:1212.5225
[38] Copi et al, Alignments from WMAP and Planck, arXiv:1311.4562v2
[39] Bennett et al, Seven Year WMAP Observations:Are there Cosmic Microwave Background Anomalies?, arXiv:1001.4758
[40] Copi et al, Large-Angle Anomalies in the CMB, arXiv:1004.5602v2
[41] The Bianchi solutions to the Einstein field equations are homogeneous but anisotropic (unlike the Standard Model which is based on the homogeneous and isotropic FLRW solution). In particular, a Bianchi Type VIIh template, which describes an anisotropic rotating Universe, can explain the anomalies, but the cosmology is unphysical – its basic parameters fail to match known cosmological data. The effect of a different kind of universe metric, one that is not homogeneous and isotropic (unlike the Standard Model), is overlaid on the underlying FLRW metric and adjusted to remove the anomalies. Such a procedure is possible, and although it reduces or eliminates the anomalies, it makes predictions that fail to match significant measured aspects of the universe.
[42] Rath et al, Direction dependence of the power spectrum and its effect on the Cosmic Microwave Background Radiation, arXiv:1302.2706v1
[43] Sarkar et al, The scale of homogeneity of the galaxy distribution in SDSS DR6, arXiv:0906.3431v1
[44] http://galileowaswrong.com/wp-content/uploads/2013/06/Planck-Date-Release.pdf
[45] http://galileowaswrong.com/wp-content/uploads/2013/11/Interview_by_Wesley_Hunt.pdf
[46] http://galileowaswrong.com/wp-content/uploads/2014/03/BICEP21.pdf
[47] The idea that the WMAP and Planck satellite experiments were motivated by a desire to prove that the CMB is not aligned with the Earth is beyond ludicrous
[48] http://magisterialfundies.blogspot.com/2013/07/new-paper-in-astronomy-and-astrophysics.html
[49] http://magisterialfundies.blogspot.co.uk/2012/09/newest-observational-evidence-shows.html
[50] http://magisterialfundies.blogspot.co.uk/2012/01/discussion-on-geocentrism.html
[51] http://www.science20.com/news_articles/planck_helps_scientists_read_cosmic_writing_wall-107174 (in the comment box)
[52] Chapter 12, Galileo Was Wrong
[53] http://magisterialfundies.blogspot.co.uk/2013/07/new-analysis-shows-universe-aligned.html
[54] In detail, the sodium and chlorine are each on a face-centred cubic arrangement, so that each atom has six nearest neighbours of the other element in a regular octahedron.
[55] Of course, they don’t have a model, a fact that we shall explore below.
[56] Peebles, and Yu, Primeval Adiabatic Perturbation in an Expanding Universe, Astrophysical Journal 162: 815–836.
[57] Doroshkevich, Zel’Dovich, and Syunyaev, Fluctuations of the microwave background radiation in the adiabatic and entropic theories of galaxy formation. In Longair and Einasto The large scale structure of the universe; Proceedings of the Symposium. Tallinn, Estonian SSR: Dordrecht, D. Reidel Publishing Co. pp. 393–404 (1977).
[58] Planck Collaboration, Planck Intermediate Results, XIII. Constraints on peculiar velocities, arXiv: 1303.5090v3
[59] http://en.wikipedia.org/wiki/Tests_of_general_relativity and http://en.wikipedia.org/wiki/Tests_of_special_relativity
[60] It’s an approximation in any case, since the universe is not homogeneous and isotropic up to a scale of at least 350 million light years but as far as the Standard Model is concerned, it’s an acceptable approximation.
[61] Zehavi, Riess, Kirshner and Dekel, A local Hubble bubble from SNe Ia, arXiv:astro-ph/9802252v2
[62] Tomita K, Distances and lensing in cosmological void models, arXiv:astro-ph/9906027v4
[63] Alnes and Amarzguioui, CMB anisotropies seen by an off-center observer in a spherically symmetric inhomogeneous universe, arXiv:astr-ph/0607334v2
[64] Moffat J W, Cosmic Microwave Background, Accelerating Universe and
Inhomogeneous Cosmology, arXiv:astro-ph/0502110v6
[65] Bellido and Haugbølle, Looking the void in the eyes – the kSZ effect in LTB models, arXiv:0807.1326v1
[66] Alnes, Amarzgiuoui and Grøn, An inhomogeneous alternative to dark energy?, arXiv:astro-ph/0512006v2
[67] Celerier M-N, The Accelerated Expansion of the Universe Challenged by an Effect of the Inhomogeneities. A Review, arXiv:astr-ph/0702416v2
See also: Alexander et al, Local Void vs Dark Energy: Confrontation with WMAP and Type Ia Supernovae, arXiv:0712.0370v3
[68] Tolman R C, Effect of inhomogeneity on cosmological models, PNAS 20, 169 (1934), http://www.ncbi.nlm.nih.gov/pmc/articles/PMC1076370/pdf/pnas01743-0027.pdf
[69] Bondi H, Spherically symmetrical models in General Relativity, Mon Not R Astron Soc 107, 410 (1947)
http://adsabs.harvard.edu/full/1947MNRAS.107..410B
[70] There are many papers on the subject of how the inhomogeneous void blends into the homogeneous background, and for a review, see, for example: Enqvist K, Lemaitre-Tolman-Bondi model and accelerating expansion, arXiv:0709.2044v1
[71] Biswas, Mansouri and Notari, Nonlinear Structure Formation and “Apparent” Acceleration: an Investigation, arXiv:astro-ph/0606703v2
[72] Scrimgeour et al, The WiggleZ Dark Energy Survey: the transition to large-scale cosmic homogeneity, arXiv:1205.6812
[73] Anderson et al, The clustering of galaxies in the SDSS-III Baryon Oscillation Spectroscopic Survey: Baryon Acoustic Oscillations in the Data Release 10 and 11 Galaxy Samples, arXiv:1312.4877v1
[74] Riess et al, A 3% Solution: Determination of the Hubble Constant with the Hubble Space Telescope and Wide Field Camera 3, Astrophysical Journal, doi:10.1088/0004-637X/730/2/119
[75] For example: Aluri and Jain, Large scale anisotropy due to pre-inflationary phase of cosmic evolution, arXiv:1108.3643v2
[76] See for example: Rubart and Schwarz, Cosmic radio dipole from NVSS and WENSS, arXiv:1301.5559v3
[77] Tiwari et al, Dipole anisotropy in sky brightness and source count distribution in radio NVSS data, arXiv:1307.1947v3
[78] Ghosh S, Generating Intrinsic Dipole Anisotropy in the Large Scale Structures, arXiv:1309.6547
[79] Rubart, Bacon and Schwarz, Impact of local structure on the cosmic radio dipole, arXiv:1402.0376
[80] Shurtleff R, A Large Scale Pattern from Optical Quasar Polarization Vectors, arXiv:1311.6118v1
[81] Hawkins, Maddox and Merrifield, No Periodicities in 2dF Redshift Survey Data, arXiv:astro-ph/0208117v1
[82] Bajan et al, On the investigations of galaxy redshift periodicity, arXiv:astro-ph/0606294
[83] The SDSS III BOSS webpage: http://www.sdss3.org/surveys/boss.php
[84] Anderson et al, The clustering of galaxies in the SDSS-III Baryon Oscillation Spectroscopic Survey: Baryon Acoustic Oscillations in the Data Release 10 and 11 Galaxy Samples, arXiv:1312.4877v1
[85] Hartnett and Hirano, Galaxy redshift abundance periodicity from Fourier analysis of number counts N(z) using SDSS and 2dF GRS galaxy surveys, arXiv:0711.4885v3
[86] Hartnett and Hirano plotted galaxy density as a pure function of redshift, rather than as a function of distance as is more commonly done
[87] Hirano and Komiya, Observational tests for oscillating expansion rate of the Universe, arXiv:1008.4456v2
[88] Bell and Comeau, More Evidence for an Oscillation Superimposed on the Hubble Flow, arXiv:1308.2624
[89] http://dealingwithcreationisminastronomy.blogspot.co.uk/2008/09/john-hartnetts-cosmos-1-introduction.html
[90] Hartnett J G, Unknown selection effect simulates redshift periodicity in quasar number counts from Sloan Digital Sky Survey, arXiv:0712.3833v3
[91] http://adsabs.harvard.edu/abs/2009ASPC..413…77H
[92] http://johnhartnett.org/2014/05/26/our-galaxy-near-the-centre-of-concentric-spherical-shells-of-galaxies/